Lung cancer biomarkers, targeted therapies and clinical assays
Introduction
Given the large heterogeneity in clinical response observed across cancer patients and the narrow therapeutic indices of anticancer drugs, novel methods for individualizing cancer therapy are critical to improve patient outcomes. Our understanding of cancer at the molecular level has resulted in a shift from characterizing tumors solely by anatomical location to consideration of their molecular profile (1). Until recently, the majority of genomic cancer research has been in discovery and validation; however, as our knowledge of tumor molecular profiling improves, genomic cancer medicine in the clinic becomes increasingly tangible (2). As the number of commercially-available clinical assays to test for tumor biomarkers increases, it is critical that clinicians understand the therapeutic implications of mutations occurring within these molecular pathways. This review aims to summarize clinically relevant cancer biomarkers, their potential relationship to lung cancer and the clinical assays available in practice to test for such biomarkers (Table 1).
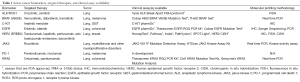
Full table
Biomarkers review
Biomarker classification
DNA analysis for pharmacogenetic purposes can be performed with either somatic or germ-line DNA. Somatic mutations are found within the tumor, requiring a tumor biopsy for identification, and are particularly useful in evaluating pharmacodynamic effects of a drug, such as tumor response. Germ-line, or inherited, variations are identified by a peripheral blood sample and help to predict the pharmacokinetic behavior of a drug, and ultimately drug response (3). Cancer biomarkers can be broadly categorized into two classifications: prognostic and predictive. A prognostic biomarker is mainly associated with disease outcome in the absence of treatment (i.e., Oncotype Dx, Mammaprint), while a predictive biomarker is valuable in assessing drug response [i.e., anaplastic lymphoma kinase (ALK), epidermal growth factor receptor (EGFR), Kirsten rat sarcoma viral oncogene homolog (KRAS)] (4). Biomarkers may also be classified as both prognostic and predictive [i.e., human epidermal growth factor receptor-2 (HER2), B-Raf proto-oncogene, serine/threonine kinase (BRAF)]. Pharmacodynamic biomarkers, a subset of predictive biomarkers, are useful in measuring the treatment effects of a drug on the tumor or on the host and can be used to guide dose selection. Examples include thiopurine-S-methyltransferase (TPMT) to guide 6-mercaptopurine dosing and uridine-diphosphate glucuronosyl transferase 1A1 (UGT1A1) to guide irinotecan dosing (5).
Lung cancer is the leading cause of cancer-related mortality worldwide. Molecularly targeted therapies have dramatically improved the ability to extend survival in patients with lung cancers positive for EGFR mutations and/or ALK translocations. Researchers in The Cancer Genome Atlas Network molecularly profiled 230 resected lung adenocarcinomas using messenger RNA, microRNA and DNA sequencing integrated with copy number, methylation and proteomic analyses. Results demonstrated high rates of mutations at a mean of 9 per megabase, while 18 genes were statistically significantly mutated including RIT1, EGFR, NF1, MET, ERBB2, RBM10, and others within the mitogen-activated protein kinase (MAPK) and phosphatidylinositol-3-kinase (PI3K) pathways (6). Although several genes identified are not currently druggable and their prognostic significance has yet to be elucidated, understanding these molecular pathways and their predictive potential are critical to advancing personalized lung cancer therapy. The remaining article will focus on cancer biomarkers for which targeted therapies are available, their influence on lung cancer therapy, and, lastly, potential new targets for drugs in the pipeline.
Cancer biomarkers and lung cancer
Anaplastic lymphoma kinase (ALK)
Activating translocations of ALK resulting in the abnormal fusion gene, EML4-ALK, occurs in approximately 2-7% of all non-small cell lung cancer (NSCLC) cases, and encodes a cytoplasmic chimeric protein with constitutive kinase activity allowing activation of the RAS-MEK-ERK, janus kinase 3 (JAK3)-STAT3, and PI3K-AKT pathways (7). Similar to EGFR mutations, ALK rearrangements in NSCLC are associated with clinical and histopathologic features, such as adenocarcinoma histology and nonsmoking history. In contrast to EGFR mutations, patients with ALK rearrangements tend to be significantly younger and male, with no significant differences in frequency between Asian and Western populations (8). Treatment with crizotinib, a tyrosine kinase inhibitor (TKI) that competitively binds to ALK, demonstrated an initial overall response rate (ORR) of 60.8% in ALK-positive NSCLC patients treated in a phase I clinical trial, advancing the molecule into an accelerated FDA approval process (7). Results from the randomized phase III trial comparing crizotinib versus docetaxel/pemetrexed in ALK-positive NSCLC unequivocally demonstrated that crizotinib results in improved ORR (65% vs. 20%; P<0.05) and median progression-free survival (PFS) (7.7 vs. 3.0 months; P<0.05) (9). Figure 1 illustrates a targeted approach to therapy selection in NSCLC based on clinically relevant biomarkers, including ALK and EGFR (discussed later in the article).
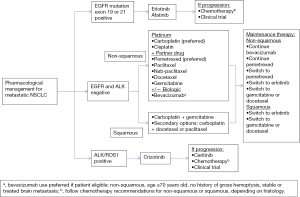
Although the majority of patients with ALK-positive NSCLC derive substantial benefit from crizotinib, this benefit is relatively short-lived secondary to acquired resistance. Possible mechanisms of resistance may include novel EGFR, KIT, MET, ROS proto-oncogene 1, receptor tyrosine kinase (ROS1) or secondary ALK mutations not previously identified (10). Ceritinib, a second generation ALK inhibitor with greater potency compared to crizotinib, received accelerated FDA approval for the treatment of metastatic ALK-positive NSCLC in patients who were previously treated with crizotinib. A phase I study demonstrated ORRs of 58% and 56% in crizotinib naïve and resistant cases, respectively (11). As evident by crizotinib and ceritinib, the drug development paradigm for highly targeted therapies is changing, allowing earlier, accelerated approval of exceedingly effective therapies, years before phase III randomized studies are completed. Additionally, companion diagnostic test approval will become increasingly common with targeted therapy approval, particularly for newly identified biomarkers [i.e., Vysis ALK Break Apart fluorescence in-situ hybridization (FISH) Probe Kit to detect ALK rearrangements].
Lastly, evidence suggests that patients with ALK-positive NSCLC have improved survival after radiotherapy for brain metastases compared with EGFR, KRAS or wild-type tumors. The median overall survival (OS) was 13.6, 26.3, 5.7 and 5.5 months in patients with EGFR, ALK, KRAS or wild-type tumors. Subsequent receipt of targeted therapy was also associated with additional improvement in OS (12).
BRAF gene
BRAF mutations have been identified in a wide range of cancers including 50% of malignant melanomas, 45% of papillary thyroid cancers, 10% of colorectal cancers, and 3% of lung cancers (13). Mutations in BRAF result in constitutive activation of downstream signaling through the MAPK pathway (14). Approximately 50-90% (depending on anatomical location) of these mutations result in the substitution of glutamic acid for valine at codon 600 (V600E) (15). In contrast to lung cancer patients with EGFR mutations and ALK rearrangements who are mostly never smokers, patients with BRAF mutations tend to be current or former smokers.
Vemurafenib, a potent and selective BRAF V600E inhibitor, and its companion diagnostic test (Cobas 4800 BRAF V600 Mutation Test) received accelerated FDA approval upon demonstrating significant improvements in OS and PFS compared to dacarbazine in metastatic melanoma patients harboring the BRAF V600E mutation [hazard ratio (HR) =0.37 for OS, HR =0.26 for PFS; P<0.001 for both] (14). Patients with BRAF-mutated colorectal tumors tend to have significantly shorter PFS and OS compared to wild-type patients, and also have the potential to impair the effects of EGFR-inhibitor therapy in KRAS wild-type patients (15). However, no benefits with vemurafenib were noted in colorectal cancer, indicating the significance of tumor origin and microenvironment (16). The data for BRAF inhibition in lung cancer is scarce, although case reports have demonstrated clinical activity with vemurafenib (complete response after 6 weeks of therapy in a patient with refractory stage IV NSCLC) (17). Another case report demonstrated clinical activity in a metastatic NSCLC patient with brain metastases, with regression of both visceral and intracranial disease (18). Interim results of a phase II study of dabrafenib in BRAF V600E-positive NSCLC patients who failed at least one line of chemotherapy showed early antitumor activity with an ORR of 54% (19).
A number of mechanisms have been elucidated for BRAF resistance, including the paradoxical activation of the MAPK pathway through RAS mutations (20). Studies have demonstrated significantly improved OS and PFS in metastatic melanoma patients receiving a concomitant mitogen-activated protein/extracellular signal-regulated kinase (MEK) inhibitor, trametinib, in combination with a selective BRAF inhibitor, dabrafenib (21). Both drugs received FDA approvals in 2013 for the treatment of patients with unresectable or metastatic melanoma with BRAF V600E or V600K mutation who have not already received a BRAF inhibitor. Similar mechanisms of resistance may be translated to lung cancer. A randomized phase II trial of docetaxel with and without the MEK inhibitor selumetinib revealed that the combination resulted in superior OS, and a statistically significant improvement in PFS and objective response rate (22). Based on promising preclinical data (23), combination of targeted therapies, such as dabrafenib plus trametinib, may ultimately prove useful in treating BRAF-positive NSCLC and should be explored further.
C-KIT gene
The C-KIT proto-oncogene encodes a receptor tyrosine kinase, which binds to stem cell factor ligand. This interaction allows for the development of melanocytes, erythrocytes, germ cells, and mast cells, ultimately resulting in dimerization, autophosporylation, and signal transduction (24). While gain-of-function C-KIT mutations are found in approximately 85% of gastrointestinal stromal tumors (GIST) and are predictive of response to imatinib therapy (25), research suggests approximately 40% of small-cell lung cancers (SCLC) overexpress C-KIT (26). However, expression of C-KIT in SCLC failed to demonstrate a significant impact as a predictive biomarker of survival, possibly due to tumor microenvironment, resulting in futility of target inhibition in this setting (26). Alternatively, evidence suggests C-KIT mutations may be a prognostic factor for worse survival (27). Current literature on C-KIT inhibition in SCLC is limited and continued researches on its prognostic and predictive value are necessary.
Epidermal growth factor receptor (EGFR)
Activating EGFR mutations result in constitutive signaling via the PI3K-AKT and RAS-MEK-ERK pathways (28). Deletions in exon 19 and a missense mutation at exon 21, resulting in an arginine to leucine substitution (L858R), account for 90% of all EGFR mutations. Approximately 15-20% of NSCLCs harbor mutated EGFR, resulting in significantly improved PFS and OS when treated with small molecule TKIs targeting the EGFR domain (erlotinib, gefitinib, afatinib) compared to traditional platinum-based chemotherapy (29). Zhou et al. prospectively tested NSCLC patients for mutated EGFR and evaluated first-line erlotinib versus chemotherapy (30). Median PFS was significantly longer in erlotinib-treated patients compared to those receiving chemotherapy (13.1 vs. 4.6 months, HR 0.16, 95% CI, 0.10-0.26; P<0.0001). The ORR was 83% and 36% for erlotinib and chemotherapy-treated patients, respectively (30). Subgroup analyses from clinical trials revealed that patients with certain clinical and histologic characteristics (female, patients of East Asian descent, non-smokers, and those with adenocarcinomas) are more likely to harbor EGFR mutations (31,32).
Currently, screening for EGFR mutations is used to select stage IV NSCLC patients that should receive erlotinib in the first-line setting. In 2013, the FDA approved a companion diagnostic test for erlotinib (Cobas EGFR Mutation Test) and authorized expanded approval for first-line use in patients with metastatic NSCLC that tests positive for the EGFR activating mutation (33). Also in 2013, a second generation EGFR inhibitor, afatinib, received FDA approval for the first-line treatment of patients with metastatic NSCLC whose tumors have EGFR mutations. Afatinib’s irreversible binding mechanism of action allows for enhanced activity in resistant tumors that have progressed after initial EGFR inhibitor therapy (34). In a phase III trial, 1,269 NSCLC patients with EGFR mutations were randomized to receive afatinib or standard chemotherapy (cisplatin and pemetrexed). The median PFS was 11.1 and 6.9 months in the afatinib and chemotherapy arms, respectively (35).
Two primary mechanisms of resistance to EGFR inhibitors include a secondary point mutation in EGFR (T790M) that blocks the capacity for erlotinib to inhibit the receptor, and the amplification of MET, which activates similar downstream signaling pathways (36). Drugs targeting EGFR T790M mutations and MET amplifications are currently under development.
Human epidermal growth factor receptor-2 (HER2)
HER2 is one of the molecular hallmarks of breast cancer and has resulted in the development of several successful targeted therapies. HER2 or ERBB2, is a member of the ERBB receptor tyrosine kinase family, which includes three additional members: EGFR (HER1/ERBB1), HER3 (ERBB3) and HER4 (ERBB4). The binding of ligands to the extracellular domain of these receptors results in dimerization, activating a catalytic cascade of events involved in cellular proliferation, differentiation and migration. HER2 status represents both a prognostic and predictive biomarker as overexpression is associated with higher breast cancer recurrence and mortality rates without consideration of pharmacological therapy; however, HER2 overexpression also predicts response to anti-HER2 targeted therapies, which has resulted in drastic improvements in median survival (37). Overexpression of HER2 may be diagnosed using immunohistochemistry (IHC) analysis (for protein expression) or FISH (for gene expression).
Trastuzumab, the first monoclonal antibody targeting the extracellular domain of HER2, was approved in 1998 as first-line treatment in combination with paclitaxel for HER2-positive advanced and metastatic breast cancer (38). Lapatinib, a small molecule TKI targeting the intracellular domain of HER2, resulted in extended survival in metastatic HER2 positive breast cancer in combination with capecitabine compared to capecitabine alone (39). Pertuzumab, an anti-HER2 humanized monoclonal antibody that inhibits receptor dimerization, prolonged PFS in metastatic breast cancer patients when combined with trastuzumab and docetaxel compared to trastuzumab and docetaxel alone (40). Trastuzumab emtansine (T-DM1), an antibody-drug conjugate combining the targeted strategy of trastuzumab with the cytotoxic properties of emtansine, prolonged PFS and OS in patients with HER2 positive, advanced BC previously treated with trastuzumab and a taxane (41).
Although HER2 overexpression and amplification has been described in 6-35% and in 10-20%, respectively, of NSCLC patients, the first clinical trials including patients treated with trastuzumab and gemcitabine-cisplatin or docetaxel, failed to demonstrate an OS benefit in HER2-positive patients (42,43). HER2 mutations have been reported to exist in approximately 1-4% of NSCLC and are more common in Asians, non-smokers, women and those with adenocarcinomas (44). Considering that HER2-positive NSCLC may benefit from HER2 inhibition or dual EGFR/HER2 inhibitions, TKIs simultaneously targeting EGFR/HER2 have been investigated. Case reports of afatinib in patients with HER2-positive NSCLC have suggested promising outcomes. Of five patients harboring HER2 mutations, three observed objective responses (45). However, studies with neratinib, an irreversible pan ERBB inhibitor, suggested no benefit in response in HER2-positive NSCLC (44). Lastly, dacomitinib, another irreversible ERBB inhibitor, has demonstrated a 14% partial response rate in HER2-positive NSCLC (46). Continued research in larger patient populations will provide a better understanding of the clinical utility of HER2 (or pan-ERBB) inhibition in HER2 positive NSCLC.
Janus kinase 2 (JAK2)
JAKs are non-receptor TKs that mediate the transmission of cytokine and growth-factor-induced intracellular signals. The mutation is a single nucleotide change, resulting in a valine to phenylalanine substitution at codon 617, and occurs in approximately 55% of patients suffering from myeloproliferative disorders (47). The transcription of numerous pro-proliferative and anti-apoptotic genes are up-regulated upon activation of the JAK-STAT pathway. Ruxolitinib is the first JAK inhibitor approved by the FDA for treatment of patients with myelofibrosis or myeloproliferative disorders. In the COMFORT-II trial, the proportion of patients achieving at least a 35% reduction in spleen volume at week 48, was 28.5% for ruxolitinib and 0% for best available therapy (P<0.0001) (48).
Although JAK mutations in NSCLC are rare, data suggests that the activation of JAK2 partially accounts for acquired erlotinib resistance. The combination of JAK2 inhibition with erlotinib in erlotinib-resistant lung cancer cell lines demonstrated restored sensitivity to erlotinib and reduction in tumor size in a murine xenograft model (49). Another study demonstrated a commonly mutated pathway in solid tumors, STAT3, is activated by JAK2 independent of other key oncogenic drivers in NSCLC; however, treatment with ruxolitinib in STAT3-activated NSCLC cell lines did not result in growth inhibition (50). Clinical trials are currently underway to investigate the influence of JAK2 inhibition with ruxolitinib in NSCLC patients receiving chemotherapy or erlotinib (ClinicalTrials.gov NCT02119650 and NCT02155465, respectively).
KRAS gene
Mutations of the KRAS oncogene have emerged as a powerful negative predictive biomarker to identify patients with metastatic colorectal cancer who do not benefit from EGFR-inhibitor therapies, such as panitumumab and cetuximab. Roughly 40% of colorectal tumors harbor a KRAS mutation (51). KRAS functions as a mediator between the extracellular ligand binding and intracellular signal transduction from the EGFR and nucleus (52). The autophosphorylation of the intracellular TK domains at codons 12 and 13 of exon 2 confers constitutive activity of downstream signaling pathways, including RAS-RAF-MAPK and PI3K-AKT pathways (51). Significant improvements in PFS were seen in KRAS wild-type colorectal cancer patients receiving EGFR-inhibitor therapy in combination with FOLFOX or FOLFIRI, while PFS was reduced in patients harboring KRAS mutations (53,54).
A meta-analysis of KRAS mutations in NSCLC described a frequency of 26% in tumors of current/former smokers, and 6% in tumors of never smokers (55). KRAS mutations have been identified as a predictor of resistance to EGFR-TKIs in NSCLC (56). While patients with KRAS mutated tumors experienced a suboptimal response to EGFR-TKIs, KRAS mutation status did not appear to affect OS (57). KRAS mutations are typically mutually exclusive of EGFR mutations and ALK translocations. While it has traditionally been extremely difficult to develop drugs to specifically target KRAS mutations, recent advances have been made to identify downstream pathways and co-mutations that indirectly affect KRAS, such as STK11 and TP53. Early research suggests that a MEK inhibitor plus docetaxel can effectively target these co-mutations. In a preclinical study, KRAS mutated mice (also mutated for STK11 and TP53) were treated with docetaxel alone or with an investigational MEK inhibitor, selumetinib (58). Concomitant loss of either TP53 or LKB1 markedly impaired the response of KRAS-mutant cancers to docetaxel monotherapy. The addition of selumetinib provided substantial benefit for mice with lung cancer caused by KRAS and KRAS-plus-TP53 mutations, though mice with co-mutations in KRAS and LKB1 were resistant to the combination. A phase II randomized trial of selumetinib plus docetaxel in KRAS-mutant NSCLC patients demonstrated a PFS of 5.3 months with the combination versus 2.1 months with docetaxel alone (P<0.05). Response rates were 37% and 0%, and median OS times were 9.4 and 5.3 months, respectively (22). Another oral MEK1/MEK2 inhibitor, trametinib, demonstrated efficacy in combination with docetaxel in KRAS-mutant and wild-type NSCLC (59). Confirmatory clinical trials are ongoing to validate the use of these agents in KRAS-mutant NSCLC.
Programmed cell death 1 (PD-1), programmed death-ligand 1 (PD-L1), PD-L2
Cancer immunotherapy rests on the premise that tumors can be recognized as foreign rather than self and can be effectively attacked by an activated immune system. However, during tumor progression, acquisition of traits that allow cancer cells to evade immune surveillance may occur by exploiting checkpoints that control the regulatory immune response (60). PD-1 receptor is an inhibitory receptor that is expressed by T cells with its ligand (PD-L1) found in the tumor microenvironment and a second ligand, PD-L2, expressed by antigen presenting cells (61). PD-L1 and PD-L2 have been shown to down-regulate T-cell activation upon binding to PD-1, especially in cancer, thus interrupting immune response (62).
Pembrolizumab is a highly selective, humanized monoclonal IgG4-kappa isotype antibody that acts against PD-1and blocks the negative immune regulatory signaling of the PD-1 receptor (61,63). Pembrolizumab has been investigated in a number of tumor types, mostly melanoma, but also NSCLC, sarcoma, carcinoid, colorectal, prostate, breast, ovarian, gastric, pancreatic and renal cell cancer (61,63-65). Grade 3 or 4 adverse events have included elevated aminotransferase, renal failure, diarrhea, hypothyroidism, fatigue, abdominal pain, decreased appetite, rash, pruritis (61). Pembrolizumab received accelerated FDA approval in September 2014 for the treatment of melanoma in patients with unresectable or metastatic disease who have disease progression following treatment with ipilimumab and, if BRAF V600 mutation positive, a BRAF inhibitor. In a phase I study of 450 NSCLC patients who had received prior chemotherapy, 159 patients had tumors with strong PD-L1 expression and received pembrolizumab 10 mg/kg IV every 3 weeks. The response rate was 23% with duration of response of 31 weeks. However, in 35 patients with tumors that were PD-L1 negative, the response rate was 9% (66). Further work is ongoing to determine the predictive nature of PD-L1 expression.
Priority review and breakthrough status was granted for nivolumab (an anti-PD-1 antibody) after investigators demonstrated significantly better response and survival outcomes with nivolumab compared to investigator’s chemotherapy in the second line treatment of patients with advanced melanoma. Subsequently, the FDA expanded the approved use to treat metastatic squamous cell NSCLC in patients who have progressed on or after platinum-based chemotherapy. In a phase I trial with expansion cohorts of 129 NSCLC patients receiving nivolumab (1 mg/kg, 3 mg/kg, or 10 mg/kg IV every 2 weeks), the ORR was 17.1% and appeared similar between squamous and non-squamous histologies. A difference in ORR between dose levels was observed: 3% for 1 mg/kg, 24.3% for 3 mg/kg and 20.3% for 10 mg/kg. The median PFS and OS were 2.3 and 9.6 months, respectively. One year after starting therapy, 42% of patients were still alive and durable responses were common with a median duration of response of 74 months (65). CheckMate-017, a phase III randomized study comparing second-line docetaxel to nivolumab (3 mg/kg) in patients with squamous cell NSCLC, was stopped early as the Data Monitoring Committee deemed that the trial had met its primary endpoint, demonstrating superior OS in patients treated with nivolumab (67). Currently, no validated marker exists to identify patients most likely to respond to anti-PD-1 therapy; however, continued investigations into the predictive value of PD-1 and PD-L1 expression is ongoing.
Investigational cancer biomarkers and lung cancer
c-MET
Signaling through the c-MET/human growth factor (HGF) pathway has been shown to trigger a variety of cellular responses, including growth, motility, metastasis, angiogenesis and tissue regeneration (68). High levels of HGF have been associated with more aggressive biology and a worse prognosis in NSCLC and SCLC. c-MET is normally expressed by epithelial cells and has been found to be overexpressed and amplified in a variety of human tumor tissues. Furthermore, the c-MET pathway is one of the key players in the development of acquired resistance to the vascular endothelial growth factor (VEGF) pathway inhibitors (68). Tumor microarray expression analysis demonstrated 72% c-MET expression in human lung cancer tissue and 40% c-MET receptor over-expression. Acquired c-MET amplification has also been linked to approximately 22% of non-T790M mediated secondary gefitinib resistance in NSCLC patients (69).
A selective c-MET inhibitor, tivantinib, has been studied in three phase I trials, either alone or in combination with erlotinib (68). The combination regimen was further studied in a phase II randomized study, which demonstrated a median PFS of 3.8 months in the combination arm versus 2.3 months in the erlotinib arm (HR 0.81, P=0.24), with no significant difference in ORR or OS (70). However, a trend towards greater benefit with the addition of tivantinib was evident in patients with c-MET positive tumors. Continued work is ongoing to further assess this agent in NSCLC. Non-selective c-MET inhibitors include crizotinib and cabozantinib. Crizotinib was initially synthesized as a c-MET inhibitor; however, after observing dramatic response in ALK-positive NSCLC, this drug essentially became recognized as an ALK inhibitor (68). Early, phase I data suggest adding cabozantinib to erlotinib is safe and effective, and is currently being explored in phase II trials. Lastly, c-MET targeted monoclonal antibodies are being studied in this setting, including onartuzumab (MetMab) (68). Phase II data suggests prolonged PFS (3.0 vs. 1.5 months; HR 0.47; P=0.01) and OS (12.6 vs. 4.6 months; HR 0.37; P=0.002) in patients with c-MET positive NSCLC receiving MetMab plus erlotinib versus erlotinib alone (71). As such, a phase III trial is ongoing to validate these findings.
Fibroblast growth factor receptor (FGFR)
The FGFR tyrosine kinase family is comprised of four kinases, FGFR1, 2, 3, and 4, that play a critical role in cell survival and tumor growth. Genetic alterations of FGFRs can lead to deregulated activation in various cancers, including breast, colorectal, bladder, in addition to lung cancer and others. A pan-FGFR TKI has been shown to block tumor proliferation in a subset of NSCLC cell lines with activated FGFR signaling but has no effect on cells that do not activate the pathway (72). A study demonstrated that FGFR1 is amplified in 21% of lung squamous cell carcinomas and 3.4% of lung adenocarcinomas (73), suggesting FGFR1 may be a potential target in mutation-positive lung cancers. In a phase I study, a selective pan-FGFR inhibitor demonstrated safety in patients with FGFR-positive squamous cell carcinoma of the lung. Early analysis demonstrated partial responses; however, robust efficacy data is not yet published (74). Another phase I trial is ongoing to assess FGFR inhibition in patients with a variety of solid tumors, including FGFR positive lung cancer (NCT01962532).
PIK3CA
The PI3K pathway is related to tumor growth in a variety of human cancers. PI3K-dependent activity is frequently elevated due to mutations of PIK3CA, the gene encoding PI3K, in addition to the loss of phosphatase and tensin homolog (PTEN) protein, a tumor suppressor with a critical role in regulating the PI3K pathway. PI3KCA activation initiates events leading to phosphorylation of Akt, which affects additional downstream signaling proteins involved in cell growth, metabolism, proliferation, survival, motility, and invasion (75). In one study, PIK3CA mutations in NSCLC were found in 3.9% of squamous cell carcinoma and 2.7% of adenocarcinoma. Furthermore, among PIK3CA mutant cases, about 50% of tumors harbored concurrent EGFR mutations and 10% had KRAS mutations. PIK3CA mutation was significantly associated with high expression of PI3K, p-Akt and mTOR, but not correlated with PIK3CA amplification. Patients with single PIK3CA mutation had shorter OS than those with PIK3CA-EGFR/KRAS co-mutation or wild-type PIK3CA (P=0.004). A significantly worse survival was also found in patients with PIK3CA mutations than those without PIK3CA mutations in the EGFR/KRAS wild-type subgroup (P=0.043), suggesting that PIK3CA mutations confer a worse prognosis (76).
A preclinical study demonstrated that targeted inhibition of PIK3CA in SCLC models harboring PI3KCA mutations resulted in cell apoptosis, inhibition of cell viability, transformation, and xenograft tumor growth, suggesting a potential role for PI3KCA inhibitors in mutated SCLC (77). Ongoing or recently completed trials in lung cancer include single-agent PI3K inhibitors (NCT01501604), as well as combinations with chemotherapy (NCT00974584, NCT00756847) (78).
Conclusions
The implementation of genomic cancer medicine relies on the foundation that genetic aberrations exist in cancer, driver oncogenic events promote mutagenesis, and these aberrations are actionable with highly targeted anticancer agents available to effectively modulate driver mutations (2). Increasing knowledge of tumor molecular profiling has led to more sophisticated treatment guidelines, such as those displayed in Figure 1. Understanding the molecular profile of tumors can help clinicians decide on the most appropriate treatment course, assist in therapeutic decision making aimed at preventing or overcoming chemoresistance, and ultimately maximize the number of effective treatment options while minimizing patients’ exposure to ineffective, yet toxic, therapies. These potential applications have resulted in a large collaboration, called Lung-MAP, among the National Cancer Institute (NCI), Southwest Oncology Group (SWOG), Friends of Cancer Research, the Foundation for the National Institutes of Health (FNIH), five pharmaceutical companies (Amgen, Genentech, Pfizer, AstraZeneca and MedImmune), and Foundation Medicine. Lung-MAP is a multi-drug, multi-arm, biomarker-driven clinical trial for patients with advanced squamous cell lung cancer (https://clinicaltrials.gov/ct2/show/NCT02154490). Real-time biopsies and diagnostic tests will identify whether patients should receive one of five therapies: an FGFR inhibitor, a PIK3CA inhibitor, a CDK4/6 inhibitor, an EGFR inhibitor, or an anti-PD-L1. A single master protocol can be amended as needed as drugs enter or exit the trial based on efficacy. Collaborative, biomarker-driven clinical trials may prove to be more clinically and cost-effective than traditional large, randomized phase III trials.
The number of pharmacogenetic assays available to identify biomarkers is continuously expanding, with several receiving accelerated FDA clearance and/or approval. The decreasing cost of assays and increasing coverage by third party payers will allow wide accessibility of these assays in clinical practice. While next generation sequencing technologies allow for the identification of a multitude of biomarkers, these technologies are not widely available in the community setting and insurance coverage remains a challenge. However, as the costs of genome sequencing continues to decline to less than $1,000, increasing demand from physicians and patients will shift routine testing from research to clinical practice, in addition to a shift from singleplex testing to multiplex sequencing. As the availability of genomic information and our knowledge of cancer at the molecular level continues to progress, clinicians must understand these intricate molecular pathways, the therapeutic implication of mutations within these pathways, and the clinical assays available to identify such biomarkers.
Acknowledgements
None.
Footnote
Conflicts of Interest: The authors have no conflicts of interest to declare.
References
- MacConaill LE, Van Hummelen P, Meyerson M, et al. Clinical implementation of comprehensive strategies to characterize cancer genomes: opportunities and challenges. Cancer Discov 2011;1:297-311. [PubMed]
- Tran B, Dancey JE, Kamel-Reid S, et al. Cancer genomics: technology, discovery, and translation. J Clin Oncol 2012;30:647-60. [PubMed]
- Deenen MJ, Cats A, Beijnen JH, et al. Part 1: background, methodology, and clinical adoption of pharmacogenetics. Oncologist 2011;16:811-9. [PubMed]
- Mandrekar SJ, Sargent DJ. Clinical trial designs for predictive biomarker validation: theoretical considerations and practical challenges. J Clin Oncol 2009;27:4027-34. [PubMed]
- Sawyers CL. The cancer biomarker problem. Nature 2008;452:548-52. [PubMed]
- Cancer Genome Atlas Research Network. Comprehensive molecular profiling of lung adenocarcinoma. Nature 2014;511:543-50. [PubMed]
- Kwak EL, Bang YJ, Camidge DR, et al. Anaplastic lymphoma kinase inhibition in non-small-cell lung cancer. N Engl J Med 2010;363:1693-703. [PubMed]
- Hancock L. The inhibition of anaplastic lymphoma kinase in non-small cell lung tumours with the ALK rearrangement may result in tumour shrinkage. Thorax 2011;66:332. [PubMed]
- Shaw AT, Kim DW, Nakagawa K, et al. Crizotinib versus chemotherapy in advanced ALK-positive lung cancer. N Engl J Med 2013;368:2385-94. [PubMed]
- Katayama R, Shaw AT, Khan TM, et al. Mechanisms of acquired crizotinib resistance in ALK-rearranged lung Cancers. Sci Transl Med 2012;4:120ra17.
- Shaw AT, Kim DW, Mehra R, et al. Ceritinib in ALK-rearranged non-small-cell lung cancer. N Engl J Med 2014;370:1189-97. [PubMed]
- Mak KS, Gainor JF, Niemierko A, et al. Significance of targeted therapy and genetic alterations in EGFR, ALK, or KRAS on survival in patients with non-small cell lung cancer treated with radiotherapy for brain metastases. Neuro Oncol 2015;17:296-302. [PubMed]
- Davies H, Bignell GR, Cox C, et al. Mutations of the BRAF gene in human cancer. Nature 2002;417:949-54. [PubMed]
- Chapman PB, Hauschild A, Robert C, et al. Improved survival with vemurafenib in melanoma with BRAF V600E mutation. N Engl J Med 2011;364:2507-16. [PubMed]
- Di Nicolantonio F, Martini M, Molinari F, et al. Wild-type BRAF is required for response to panitumumab or cetuximab in metastatic colorectal cancer. J Clin Oncol 2008;26:5705-12. [PubMed]
- Prahallad A, Sun C, Huang S, et al. Unresponsiveness of colon cancer to BRAF(V600E) inhibition through feedback activation of EGFR. Nature 2012;483:100-3. [PubMed]
- Peters S, Michielin O, Zimmermann S. Dramatic response induced by vemurafenib in a BRAF V600E-mutated lung adenocarcinoma. J Clin Oncol 2013;31:e341-4. [PubMed]
- Robinson SD, O'Shaughnessy JA, Cowey CL, et al. BRAF V600E-mutated lung adenocarcinoma with metastases to the brain responding to treatment with vemurafenib. Lung Cancer 2014;85:326-30. [PubMed]
- Planchard D, Mazieres J, Riely GJ, et al. Interim results of phase II study BRF113928 of dabrafenib in BRAF V600E mutation–positive non-small cell lung cancer (NSCLC) patients. J Clin Oncol 2013;31:abstr 8009.
- Su F, Viros A, Milagre C, et al. RAS mutations in cutaneous squamous-cell carcinomas in patients treated with BRAF inhibitors. N Engl J Med 2012;366:207-15. [PubMed]
- Flaherty KT, Infante JR, Daud A, et al. Combined BRAF and MEK inhibition in melanoma with BRAF V600 mutations. N Engl J Med 2012;367:1694-703. [PubMed]
- Jänne PA, Shaw AT, Pereira JR, et al. Selumetinib plus docetaxel for KRAS-mutant advanced non-small-cell lung cancer: a randomised, multicentre, placebo-controlled, phase 2 study. Lancet Oncol 2013;14:38-47. [PubMed]
- Joshi M, Rice SJ, Liu X, et al. Trametinib with or without vemurafenib in BRAF mutated non-small cell lung cancer. PLoS One 2015;10:e0118210. [PubMed]
- Yasuda A, Sawai H, Takahashi H, et al. Stem cell factor/c-kit receptor signaling enhances the proliferation and invasion of colorectal cancer cells through the PI3K/Akt pathway. Dig Dis Sci 2007;52:2292-300. [PubMed]
- Heinrich MC, Corless CL, Demetri GD, et al. Kinase mutations and imatinib response in patients with metastatic gastrointestinal stromal tumor. J Clin Oncol 2003;21:4342-9. [PubMed]
- Boldrini L, Ursino S, Gisfredi S, et al. Expression and mutational status of c-kit in small-cell lung cancer: prognostic relevance. Clin Cancer Res 2004;10:4101-8. [PubMed]
- Lu HY, Zhang G, Cheng QY, et al. Expression and mutation of the c-kit gene and correlation with prognosis of small cell lung cancer. Oncol Lett 2012;4:89-93. [PubMed]
- Yarden Y, Shilo BZ. SnapShot: EGFR signaling pathway. Cell 2007;131:1018. [PubMed]
- Lynch TJ, Bell DW, Sordella R, et al. Activating mutations in the epidermal growth factor receptor underlying responsiveness of non-small-cell lung cancer to gefitinib. N Engl J Med 2004;350:2129-39. [PubMed]
- Zhou C, Wu YL, Chen G, et al. Erlotinib versus chemotherapy as first-line treatment for patients with advanced EGFR mutation-positive non-small-cell lung cancer (OPTIMAL, CTONG-0802): a multicentre, open-label, randomised, phase 3 study. Lancet Oncol 2011;12:735-42. [PubMed]
- Thatcher N, Chang A, Parikh P, et al. Gefitinib plus best supportive care in previously treated patients with refractory advanced non-small-cell lung cancer: results from a randomised, placebo-controlled, multicentre study (Iressa Survival Evaluation in Lung Cancer). Lancet 2005;366:1527-37. [PubMed]
- Shepherd FA, Rodrigues Pereira J, Ciuleanu T, et al. Erlotinib in previously treated non-small-cell lung cancer. N Engl J Med 2005;353:123-32. [PubMed]
- US Food and Drug Administration. Table of Pharmacogenomic Biomarkers in Drug Labels. Available online: http://www.fda.gov/Drugs/ScienceResearch/ResearchAreas/Pharmacogenetics/ucm083378.htm, accessed 2 April 2015.
- Miller VA, Hirsh V, Cadranel J, et al. Afatinib versus placebo for patients with advanced, metastatic non-small-cell lung cancer after failure of erlotinib, gefitinib, or both, and one or two lines of chemotherapy (LUX-Lung 1): a phase 2b/3 randomised trial. Lancet Oncol 2012;13:528-38. [PubMed]
- Sequist LV, Yang JC, Yamamoto N, et al. Phase III study of afatinib or cisplatin plus pemetrexed in patients with metastatic lung adenocarcinoma with EGFR mutations. J Clin Oncol 2013;31:3327-34. [PubMed]
- Califano R, Morgillo F, De Mello RA, et al. Role of mesenchymal-epithelial transition amplification in resistance to anti-epidermal growth factor receptor agents. Ann Transl Med 2015;3:81. [PubMed]
- Press MF, Bernstein L, Thomas PA, et al. HER-2/neu gene amplification characterized by fluorescence in situ hybridization: poor prognosis in node-negative breast carcinomas. J Clin Oncol 1997;15:2894-904. [PubMed]
- Romond EH, Perez EA, Bryant J, et al. Trastuzumab plus adjuvant chemotherapy for operable HER2-positive breast cancer. N Engl J Med 2005;353:1673-84. [PubMed]
- Geyer CE, Forster J, Lindquist D, et al. Lapatinib plus capecitabine for HER2-positive advanced breast cancer. N Engl J Med 2006;355:2733-43. [PubMed]
- Baselga J, Cortés J, Kim SB, et al. Pertuzumab plus trastuzumab plus docetaxel for metastatic breast cancer. N Engl J Med 2012;366:109-19. [PubMed]
- Verma S, Miles D, Gianni L, et al. Trastuzumab emtansine for HER2-positive advanced breast cancer. N Engl J Med 2012;367:1783-91. [PubMed]
- Krug LM, Miller VA, Patel J, et al. Randomized phase II study of weekly docetaxel plus trastuzumab versus weekly paclitaxel plus trastuzumab in patients with previously untreated advanced nonsmall cell lung carcinoma. Cancer 2005;104:2149-55. [PubMed]
- Gatzemeier U, Groth G, Butts C, et al. Randomized phase II trial of gemcitabine-cisplatin with or without trastuzumab in HER2-positive non-small-cell lung cancer. Ann Oncol 2004;15:19-27. [PubMed]
- Garrido-Castro AC, Felip E. HER2 driven non-small cell lung cancer (NSCLC): potential therapeutic approaches. Transl Lung Cancer Res 2013;2:122-7. [PubMed]
- De Grève J, Teugels E, Geers C, et al. Clinical activity of afatinib (BIBW 2992) in patients with lung adenocarcinoma with mutations in the kinase domain of HER2/neu. Lung Cancer 2012;76:123-7. [PubMed]
- Carpenter RL, Lo HW. Dacomitinib, an emerging HER-targeted therapy for non-small cell lung cancer. J Thorac Dis 2012;4:639-42. [PubMed]
- Kilpivaara O, Mukherjee S, Schram AM, et al. A germline JAK2 SNP is associated with predisposition to the development of JAK2(V617F)-positive myeloproliferative neoplasms. Nat Genet 2009;41:455-9. [PubMed]
- Ostojic A, Vrhovac R, Verstovsek S. Ruxolitinib for the treatment of myelofibrosis: its clinical potential. Ther Clin Risk Manag 2012;8:95-103. [PubMed]
- Harada D, Takigawa N, Ochi N, et al. JAK2-related pathway induces acquired erlotinib resistance in lung cancer cells harboring an epidermal growth factor receptor-activating mutation. Cancer Sci 2012;103:1795-802. [PubMed]
- Looyenga BD, Hutchings D, Cherni I, et al. STAT3 is activated by JAK2 independent of key oncogenic driver mutations in non-small cell lung carcinoma. PLoS One 2012;7:e30820. [PubMed]
- Siddiqui AD, Piperdi B. KRAS mutation in colon cancer: a marker of resistance to EGFR-I therapy. Ann Surg Oncol 2010;17:1168-76. [PubMed]
- Ono M, Kuwano M. Molecular mechanisms of epidermal growth factor receptor (EGFR) activation and response to gefitinib and other EGFR-targeting drugs. Clin Cancer Res 2006;12:7242-51. [PubMed]
- Douillard JY, Siena S, Cassidy J, et al. Randomized, phase III trial of panitumumab with infusional fluorouracil, leucovorin, and oxaliplatin (FOLFOX4) versus FOLFOX4 alone as first-line treatment in patients with previously untreated metastatic colorectal cancer: the PRIME study. J Clin Oncol 2010;28:4697-705. [PubMed]
- Peeters M, Price TJ, Cervantes A, et al. Randomized phase III study of panitumumab with fluorouracil, leucovorin, and irinotecan (FOLFIRI) compared with FOLFIRI alone as second-line treatment in patients with metastatic colorectal cancer. J Clin Oncol 2010;28:4706-13. [PubMed]
- Dearden S, Stevens J, Wu YL, et al. Mutation incidence and coincidence in non small-cell lung cancer: meta-analyses by ethnicity and histology (mutMap). Ann Oncol 2013;24:2371-6. [PubMed]
- Linardou H, Dahabreh IJ, Bafaloukos D, et al. Somatic EGFR mutations and efficacy of tyrosine kinase inhibitors in NSCLC. Nat Rev Clin Oncol 2009;6:352-66. [PubMed]
- Massarelli E, Varella-Garcia M, Tang X, et al. KRAS mutation is an important predictor of resistance to therapy with epidermal growth factor receptor tyrosine kinase inhibitors in non-small-cell lung cancer. Clin Cancer Res 2007;13:2890-6. [PubMed]
- Chen Z, Cheng K, Walton Z, et al. A murine lung cancer co-clinical trial identifies genetic modifiers of therapeutic response. Nature 2012;483:613-7. [PubMed]
- Gandara DR, Hiret S, Blumenschein GR, et al. Oral MEK1/MEK2 inhibitor trametinib (GSK1120212) in combination with docetaxel in KRAS-mutant and wild-type (WT) advanced non-small cell lung cancer (NSCLC): A phase I/Ib trial. J Clin Oncol 2013;31:abstr 8028.
- Greenwald RJ, Freeman GJ, Sharpe AH. The B7 family revisited. Annu Rev Immunol 2005;23:515-48. [PubMed]
- Hamid O, Robert C, Daud A, et al. Safety and tumor responses with lambrolizumab (anti-PD-1) in melanoma. N Engl J Med 2013;369:134-44. [PubMed]
- Freeman GJ, Long AJ, Iwai Y, et al. Engagement of the PD-1 immunoinhibitory receptor by a novel B7 family member leads to negative regulation of lymphocyte activation. J Exp Med 2000;192:1027-34. [PubMed]
- Patnaik A, Kang SP, Tolcher AW, et al. Phase I study of MK-3475 (anti-PD-1 monoclonal antibody) in patients with advanced solid tumors. J Clin Oncol 2012;30:abstr 2512.
- Brahmer JR, Tykodi SS, Chow LQ, et al. Safety and activity of anti-PD-L1 antibody in patients with advanced cancer. N Engl J Med 2012;366:2455-65. [PubMed]
- Topalian SL, Hodi FS, Brahmer JR, et al. Safety, activity, and immune correlates of anti-PD-1 antibody in cancer. N Engl J Med 2012;366:2443-54. [PubMed]
- Garon E, Leighl NB, Rizvi NA, et al. Safety and clinical activity of MK-3475 in previously treated patients (pts) with non-small cell lung cancer (NSCLC). J Clin Oncol 2014;32:abstr 8020.
- Bristol-Myers Squibb. CheckMate -017, A Phase 3 Study of Opdivo (Nivolumab) Compared to Docetaxel in Patients with Second-Line Squamous Cell Non-small Cell Lung Cancer, Stopped Early. Available online: http://news.bms.com/press-release/checkmate-017-phase-3-study-opdivo-nivolumab-compared-docetaxel-patients-second-line-s, accessed 2 April 2015.
- Menis J, Giaj Levra M, Novello S. MET inhibition in lung cancer. Transl Lung Cancer Res 2013;2:23-39. [PubMed]
- Ma PC, Tretiakova MS, MacKinnon AC, et al. Expression and mutational analysis of MET in human solid cancers. Genes Chromosomes Cancer 2008;47:1025-37. [PubMed]
- Sequist LV, von Pawel J, Garmey EG, et al. Randomized phase II study of erlotinib plus tivantinib versus erlotinib plus placebo in previously treated non-small-cell lung cancer. J Clin Oncol 2011;29:3307-15. [PubMed]
- Spigel DR, Ervin TJ, Ramlau R, et al. Final efficacy results from OAM4558g, a randomized phase II study evaluating MetMAb or placebo in combination with erlotinib in advanced NSCLC. J Clin Oncol 2011;29:abstr 7505.
- Marek L, Ware KE, Fritzsche A, et al. Fibroblast growth factor (FGF) and FGF receptor-mediated autocrine signaling in non-small-cell lung cancer cells. Mol Pharmacol 2009;75:196-207. [PubMed]
- Dutt A, Ramos AH, Hammerman PS, et al. Inhibitor-sensitive FGFR1 amplification in human non-small cell lung cancer. PLoS One 2011;6:e20351. [PubMed]
- Sequist LV, Cassier P, Varga A, et al. Phase I study of BGJ398, a selective pan-FGFR inhibitor in genetically preselected advanced solid tumors. 2014 AACR Annual Meeting. San Diego, USA, 2014:abstract CT326.
- Vogt PK, Bader AG, Kang S. Phosphoinositide 3-kinase: from viral oncoprotein to drug target. Virology 2006;344:131-8. [PubMed]
- Wang L, Hu H, Pan Y, et al. PIK3CA mutations frequently coexist with EGFR/KRAS mutations in non-small cell lung cancer and suggest poor prognosis in EGFR/KRAS wildtype subgroup. PLoS One 2014;9:e88291. [PubMed]
- Walls M, Baxi SM, Mehta PP, et al. Targeting small cell lung cancer harboring PIK3CA mutation with a selective oral PI3K inhibitor PF-4989216. Clin Cancer Res 2014;20:631-43. [PubMed]
- Oxnard GR, Binder A, Jänne PA. New targetable oncogenes in non-small-cell lung cancer. J Clin Oncol 2013;31:1097-104. [PubMed]