Sex dimorphism in response to targeted therapy and immunotherapy in non-small cell lung cancer patients: a narrative review
Introduction
The American cancer statistics for the year 2021 ranks lung cancer as the second most common (number of new cases) in men and women and attributes it to one-quarter of all cancer death (1). The majority (85%) of lung cancer patients have non-small cell lung cancer (NSCLC) (2). Despite the alarming statistics, the arrival of targeted therapy and immunotherapy has each been a major breakthrough in improving the NSCLC survival rates. For instance, annual decrease in NSCLC incidence-based mortality has markedly dropped after 2013, the year erlotinib was approved by the U.S. FDA as a first-line therapy for metastatic NSCLC with exon 19 deletion or exon 21 L858R substitution (3). The latest evolution in the NSCLC treatment landscape has been immunotherapy, and the research and development efforts surrounding this treatment strategy are currently ongoing.
A major challenge for these new classes of treatment is the large inter-patient variability in therapeutic response, which highlights the need for better biologic or clinical markers that can improve the ability of clinicians to predict patient response. Among various potential patient factors, sex stands out as an easy-to-use clinical characteristic that can reflect the genetic, epigenetic, and environmental effects influencing the patient (4). Additionally, response to drugs have been reported to differ based on sex (5,6). Therefore, we survey the knowledge in sex difference in NSCLC treatment response. Differences in tumoral features have been found between men and women alongside the difference of incidence, prognosis, and mortality in NSCLC. For instance, women are more likely than men to develop adenocarcinoma while men have higher rates of squamous cell carcinoma and large-cell lung cancer (7-10). Similarly, studies have found sex-based differences in common driver mutations of NSCLC (11,12). The underlying causes of these differences can generally be attributed to internal factors such as genetics, hormone, and immune system, as well as environmental factors such as social behaviors (e.g., smoking) (Figure 1). Given that NSCLC is not entirely the same disease between men and women, a better understanding of differences in response to treatment based on patient sex has high potential to improve patient outcome in the future (13). Regulatory authorities’ recent efforts to encourage sex-based assessments in clinical trials have produced limited results so far (14). In order to better orient future studies of newer anticancer agents to sex-based personalization of therapies, it is worthwhile to review the existing literature on sex-based difference in the effectiveness of the existing targeting and immunotherapy agents against NSCLC.
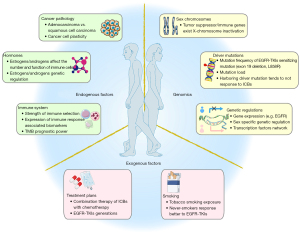
In this review, we summarize and discuss reported sex differences in treatment effects (focusing on survival outcomes) in NSCLC patients. We focus on targeted therapy and immunotherapy and outline the evidence on sex differences in response to these treatments in clinical trials. We describe the current knowledge on sex dimorphism in genetic, immunologic, and behavioral factors that may impact the treatment outcomes. With this review, we aim to stimulate further research on the impact of sex as a biological variable on treatment response, with the goal of improving clinical understanding and decision making, We present the following article in accordance with the Narrative Review reporting checklist (available at https://tlcr.amegroups.com/article/view/10.21037/tlcr-21-1013/rc).
Methods
We searched published meta-analysis/systematic reviews in PubMed for the following approved targeting therapy and immune checkpoint blockade (ICB) agents in NSCLC. For targeting therapy, we focused on agents that are targeting epidermal growth factor receptor (EGFR), angiogenesis, Kirsten rat sarcoma virus (KRAS), anaplastic lymphoma kinase (ALK), c-ros oncogene 1 (ROS1), B-Raf Proto-Oncogene (BRAF), Ret Proto-Oncogene (RET), MET Proto-Oncogene (MET), Neurotrophic tyrosine receptor kinase (NTRK) (The name of each agent was retrieved from https://www.cancer.org/cancer/lung-cancer/treating-non-small-cell/targeted-therapies.html); for ICB, we focused on agents that target “Programmed cell death protein 1 (PD1)”, “Programmed death-ligand 1 (PD-L1)”, and “Cytotoxic T-Lymphocyte Associated Protein 4 (CTLA4)”. Subsequently, we manually screened the resulting articles for “sex difference”, “sex differences”, “gender differences” in the title/abstract area to narrow down the articles to be included in our review (Table 1).
Table 1
Items | Specification |
---|---|
Date of search | No time restriction |
Databases and other sources searched | the PubMed website |
Search terms used (including MeSH and free text search terms and filters) | Searching terms were described above. A complete searching terms is listed in Table S1 |
Timeframe | No time restriction |
Inclusion and exclusion criteria | Meta-analysis/systematic reviews; no language restriction |
Selection process | The co-first authors conducted the conducted the selection independently. |
Any additional considerations, if applicable | N/A |
NSCLC, non-small cell lung cancer; MeSH, Medical Subject Headings.
Sex differences in response to targeted therapy in NSCLC
Epidermal growth factor receptor tyrosine kinase inhibitors (EGFR TKIs)
The epidermal growth factor receptor (EGFR) is a transmembrane receptor whose activation of downstream signal pathways is involved in several key cellular functions including proliferation, differentiation, and survival (15). Driver mutations that activate or overexpress EGFR have been associated with NSCLC as major therapeutic targets (16,17). Among these, exon 19 deletion and L858R substitution in exon 21 are some of the most common examples.
Gefitinib and erlotinib are the first-generation small molecule EGFR tyrosine kinase inhibitors (TKIs) that block EGFR’s signaling pathway by competitively binding at the ATP site of its tyrosine kinase domain (18). Both have been approved by the FDA for metastatic NSCLC positive for exon 19 deletion or exon 21 substitution.
Afatinib and dacomitinib are the second-generation EGFR TKIs. Afatinib inhibits the tyrosine kinase domain of ErbB family of receptors (EGFR 1, ErbB2, and ErbB4), and therefore has a wider range of target receptors compared to the first-generation agents (19). Dacomitinib likewise has shown to have inhibitory activity against EGFR, ErbB2, and ErbB4 (20). These irreversible covalent inhibitors of EGFR are active against cancers resistant to erlotinib or gefitinib except those with T790M substitution, a major resistance mutation for first and second generation EGFR-TKIs (21).
Osimertinib, a third-generation EGFR-TKI, has been FDA-approved for metastatic NSCLC positive for T790M substitution as well as exon 19 deletion or exon 21 L858R. It is also currently the only preferred agent for treating EGFR mutation positive NSCLC in the National Comprehensive Cancer Network (NCCN) guidelines (22). The third generation EGFR TKI has been developed largely to target T790M substitution positive cancers.
Females tend to have better PFS than males in receiving first/second generation EGFR TKIs
Sex differences in response to first generation EGFR-TKIs have been noted in the literature. Chen et al. performed a meta-analysis using five randomized controlled trials of first-generation EGFR TKIs as maintenance therapy in comparison with placebo control for stage IIIB/IV NSCLC (two studies with gefitinib and three with erlotinib; a total of 2,436 patients included) (23). The analysis showed that maintenance using first generation EGFR-TKIs provided benefit to both sexes in progression free survival [male: hazard ratio (HR) of progression free survival (PFS) =0.68, 95% CI: 0.55–0.82; female: HR of PFS =0.52, 95% CI: 0.37–0.68] while only females showed statistically significant overall survival (OS) benefits (male: HR of OS =0.91, 95% CI: 0.79–1.03; female: HR of OS =0.73, 95% CI: 0.58–0.89).
Pinto et al. performed another meta-analysis on six phase III trials that compared gefitinib, erlotinib, or afatinib to chemotherapy in order to assess any sex-based difference in response to these agents (total 1,425 patients; 931 females and 494 males) (24). While patients of both sexes had statistically significant PFS benefit from EGFR-TKI therapies, the investigators evaluated the size of PFS benefit for each sex and reported that females showed greater PFS benefit (HR of PFS =0.34, 95% CI: 0.28–0.40) than males did (HR of PFS =0.44, 95% CI: 0.34–0.56). However, no interaction test was reported to determine the statistical significance of this observed difference. Another meta-analysis with seven trials (six of which were included in the abovementioned meta-analysis) conducted by Hasegawa et al. demonstrated a female-bias in benefits from EGFR-TKI treatments (female: HR of PFS =0.31, 95% CI: 0.23–0.40; male: HR of PFS =0.43, 95% CI: 0.32–0.57) (25). However, a meta-regression analysis conducted by the investigators showed that the observed difference in PFS benefit between the two sexes was not significant (P=0.09).
More recently, a meta-analysis performed by Xiao et al. assessed the efficacy differences of EGFR-TKIs (6 studies on gefitinib, 12 on erlotinib, 2 on afatinib, 1 on dacomitinib, and 1 on icotinib) in OS by sex and smoking status (26). The primary analysis of pooled interaction HRs of sex did not find any significant differences in OS between males and females. However, in the subgroup analysis, women showed significantly greater OS benefit compared to men when EGFR-TKIs were compared to placebo (HR of interaction =0.86, 95% CI: 0.75–1.00). No significant OS difference was observed between sexes when EGFR-TKIs were compared to chemotherapy. Similarly, no sex differences in OS were observed in any other subgroups (e.g., subgroups of specific agents, different lines of therapy, and different EGFR mutation statuses). The investigators have attributed this difference to previous findings that suggest better OS response of women to chemotherapy compared to men (27). This suggests that placebo-controlled results should be free of the sex-related confounding effect of chemotherapy. In other words, the subgroup analysis of placebo-controlled results may be considered more representative of the actual sex-based difference in OS response to EGFR-TKIs.
While two of the meta-analyses discussed above include afatinib trials, no meta-analysis has been found to the best of our abilities that specifically looks at sex-based difference in response to any one or both of the second-generation agents. One small retrospective study by Wang et al. evaluated 60 Chinese patients with advanced NSCLC with sensitive EGFR mutations who received afatinib treatments (28). The investigators found using multivariate cox proportional regression analyses that sex did not significantly affect the PFS of the studied patients regardless of the line of therapy.
Table 2 provides a summary of the meta-analyses included in this review. Overall, a female-favoring trend of sex difference has been observed in NSCLC patients receiving first and second-generation EGFR-TKIs when the treatment effectiveness is evaluated by PFS. Although the significance of the difference has not been evaluated in most of the included studies, the trend appears to be consistent. PFS is more likely to reflect the immediate response to treatment. Therefore, it would be reasonable to suggest that female tend to have a better response to the EGFR-TKIs than male. This trend in benefit difference has not been consistently observed in terms of OS, which reflects a combination of all treatment effects and patient characteristics.
Table 2
Study | Total patients | Treatment | Control | Overall pooled HR of PFS (95% CI) | Overall pooled HR of OS (95% CI) | Sex of pooled HR of PFS (95% CI) | Sex of pooled HR of OS (95% CI) | Interaction HR of sex (PFS, 95% CI) | Interaction HR of sex (OS, 95% CI) | |||
---|---|---|---|---|---|---|---|---|---|---|---|---|
Female | Male | Female | Male | |||||||||
EGFR-TKIs | ||||||||||||
(29) | 1,942 | Erlotinib | Standard chemotherapy | 0.76 (0.70, 0.83) | 0.87 (0.80, 0.95) | 0.65 (0.55, 0.77) | 0.80 (0.73, 0.88) | NR | NR | NR | NR | |
(23) | 2,436 | Erlotinib or gefitinib | Placebo | 0.63 (0.50, 0.76) | 0.84 (0.76, 0.93) | 0.52 (0.37, 0.68) | 0.68 (0.55, 0.82) | 0.73 (0.58, 0.89) | 0.91 (0.79, 1.03) | NR | NR | |
(30) | 1,649 | Erlotinib or gefitinib or afatinib | Standard chemotherapy | 0.37 (0.32, 0.42) | NR | 0.33 (0.28, 0.38) | 0.45 (0.36, 0.55) | NR | NR | P=0.03 | NR | |
(31) | 1,231 | Erlotinib or gefitinib | Standard chemotherapy | 0.37 (0.32, 0.42) | 1.01 (0.88, 1.17) | 0.34 (0.29, 0.41) | 0.42 (0.33, 0.54) | 1.02 (0.86, 1.21) | 0.98 (0.76, 1.27) | NR | NR | |
(32) | 975 | Osimertinib | Erlotinib or gefitinib/standard chemotherapy | 0.38 (0.29, 0.50) | 0.66 (0.48, 0.89) | 0.37 (0.30, 0.46) | 0.51 (0.39, 0.67) | NR | NR | NR | NR | |
(26) | 11,154 | Erlotinib or gefitinib or afatinib or icotinib | Placebo or chemotherapy | NR | 0.94 (0.89, 1.00) | NR | NR | NR | NR | NR | 0.95 (0.87, 1.04) | |
(24) | 1,425 | Erlotinib or gefitinib or afatinib | chemotherapy | NR | NR | 0.34 (0.28, 0.40) | 0.44 (0.34, 0.56) | NR | NR | NR | NR | |
(25) | 1,649 | Erlotinib or gefitinib or afatinib | Platinum-doublet chemotherapy | NR | NR | 0.31 (0.23, 0.40) | 0.43 (0.32, 0.57) | NR | NR | Meta-regression of HRs: P=0.090 | NR | |
ICBs | ||||||||||||
(33) | 3,144 | PD-1/PD-L1 inhibitor plus chemotherapy | Standard chemotherapy | 0.62 (0.57, 0.67) | 0.68 (0.53, 0.87) | 0.60 (0.44, 0.81) | 0.65 (0.58, 0.74) | 0.32 (0.23, 0.46) | 0.69 (0.55, 0.87) | P=0.365 | P<0.001 | |
(34) | 6,964 | PD-1/PD-L1 inhibitor/CTLA-4 inhibitors plus chemotherapy | Standard chemotherapy | NR | NR | NR | NR | 0.89 (0.71, 1.11) | 0.72 (0.61, 0.86) | NR | P=0.72 | |
(35) | 6,645 | PD-1/PD-L1 inhibitor/CTLA-4 inhibitors plus chemotherapy | Standard chemotherapy | NR | NR | NR | NR | 0.72 (0.56, 0.93) | 0.79 (0.71, 0.88) | NR | P=0.79 | |
(36) | 4,923 (PFS) | PD-1/PD-L1 inhibitor plus chemotherapy | Standard chemotherapy | NR | NR | 0.56 (0.49, 0.65) | 0.64 (0.56, 0.71) | 0.48 (0.35, 0.67) | 0.76 (0.66, 0.87) | 1.15 (0.96, 1.38) | 1.56 (1.21, 2.01) | |
2,970 (OS) | Favors greater effect of treatment in women | Favors greater effect of treatment in women | ||||||||||
(36) | 2,120 | PD-1 inhibitors | Standard chemotherapy | NR | NR | NR | NR | 0.78 (0.60, 1.01) | 0.97 (0.79, 1.19) | NR | 0.83 (0.65, 1.06) | |
Favors greater effect of treatment in men | ||||||||||||
(37) | 3,867 | PD-1/PD-L1 inhibitors | Standard chemotherapy | 0.84 (0.72, 0.97) | 0.72 (0.63, 0.82) | 1.02 (0.84, 1.23) | 0.72 (0.55, 0.93) | 0.76 (0.62, 0.93) | 0.74 (0.63, 0.87) | NR | NR | |
(38) | 3,025 | PD-1/PD-L1 inhibitors | Docetaxel | NR | 0.69 (0.63, 0.75) | NR | NR | 0.70 (0.60, 0.82) | 0.69 (0.61, 0.77) | NR | P=0.82 | |
(39) | 1,672 | PD-1/PD-L1 inhibitors | Platinum-based chemotherapy | NR | NR | NR | NR | 0.84 (0.64, 1.10) | 0.59 (0.50, 0.69) | NR | P=0.04 |
HR, hazard ratio; NR, not reported; PD-1, programmed cell death protein 1; PD-L1, programmed death-ligand 1; OS, overall survival; PFS, progression free survival; EGFR, epidermal growth factor receptor; TKI, tyrosine kinase inhibitor; ICB, immune checkpoint blockade; NSCLC, non-small cell lung cancer.
No sex differences in response to third generation EGFR TKIs
Current existing literature does not support sex-based differences in patient response to the third generation EGFR TKIs. Huang et al. performed a meta-analysis assessing the efficacy of osimertinib on EGFR mutation-positive advanced NSCLC against that of previous generation EGFR TKIs or chemotherapy (32). Analyzing 975 patients over two trials, the investigators showed that, while women (HR of PFS =0.37, 95% CI: 0.30–0.46) had a trend of better response compared to men (HR of PFS =0.51, 95% CI: 0.39–0.67), there was no statistically significant difference (male vs. female; P=0.063).
Female sex is associated with higher frequency of EGFR alterations but this observation is confounded by other factors
Sex differences of EGFR alterations have been observed in NSCLC patients. Comprehensive analyses from The Cancer Genome Atlas (TCGA) on the molecular level reveal higher mutation frequency and gene expression of EGFR in female lung adenocarcinoma (LUAD) patients after controlling for other clinical features (40). This may lead to a higher response rate to the first-generation EGFR-TKIs in female patients. Moreover, there have been several studies showing the potential interplay between patient characteristics and EGFR mutations. Midha et al. conducted a comprehensive review of the frequency of EGFR mutations in patients with LUAD across 14 countries and found that the frequency of EGFR mutations was higher in women in all regions analyzed except Bangladesh (11). However, the investigators did not report on individual frequencies of specific mutations for EGFR. Similarly, Zhang et al. conducted a meta-analysis which revealed higher prevalence of EGFR mutations (mostly in exon 19 or 21) in female Caucasian (OR is 2.7, 95% CI: 2.3–3.3) and Asian (OR is 2.8, 95% CI: 2.6–3.1) populations (12). However, no sex-based estimation of the prevalence of specific mutations was provided. A similar observation has been reported in East Asian patients: females show higher frequencies of EGFR mutations while males show higher frequencies of KRAS and tumor protein P53 (TP53) mutations (41).
In addition to ethnicity, Sugio et al. showed that, in patients with LUAD, female sex and never-smoker status were associated with higher incidence of EGFR mutations (exon 19 deletion or exon 21 L858R substitution) (42). Additionally, exon 21 L858R was significantly more frequent in females and never-smokers. Tanaka et al. showed that female sex, smoking history of less than 20 pack-per-year (including never-smokers), and adenocarcinoma or adeno-squamous cell carcinoma histology subgroup were associated with higher frequency of EGFR mutations (mainly exon 19 deletion and exon 21 L858R) (43). However, when the confounders were accounted for using logistic regression, the investigators found that histology (adenocarcinoma or adeno-squamous cell carcinoma) and smoking history (less than 20 packs per year), but not female sex, were associated with frequency of EGFR mutations. Hsiao et al. found similar results when they conducted a logistic regression to analyze age, sex, smoking status, histology, and cancer stage as predictors of EGFR mutations (exon 19 deletion or exon 21 L858R) (44). Univariate analysis showed higher OR for EGFR mutation with female sex, never smoker, and adenocarcinoma. However, only never smoker and adenocarcinoma (but not sex) were shown to be predictors of EGFR mutation frequency in multivariate analysis. Collectively, even though female sex has been reported to be associated with higher EGFR mutation frequency, this association may be the results of the interaction between sex and other confounding clinical characteristics such as smoking status and disease pathology. T790M substitution is a biomarker of resistance to the first and second-generation EGFR-TKIs. Several retrospective studies that analyzed biopsy samples from NSCLC patients revealed no significant sex differences in the frequency of T790M substitution (45-49). Aside from T790M mutation, other genetic alterations, such as EGFRC797X, are reasons for drug resistance against EGFR-TKIs (50). However, relevant analysis on any potential sex differences in the frequencies of these alterations is lacking.
Smoking status and sex are independent predictors to the treatment effectiveness of EGFR TKIs
Since current literature supports association of never-smoker status and higher frequency of mutations in exons 18–21 among NSCLC patients, we sought to assess any interplay between smoking status and sex on their impact on EGFR-TKIs efficacy (51,52). Independent of sex, never smokers have been shown to derive significantly greater PFS benefits from EGFR-TKIs compared to smokers (P=0.007), although this difference has not been observed in OS (25,26). Lee et al. performed multivariable analyses on four clinical trials to investigate the potential interplay among three patient characteristics (sex, smoking status, and EGFR mutation) on their effects on PFS benefit from EGFR TKI therapy (30). Patients with each of the following characteristics had greater PFS benefit from EGFR TKIs compared to those with counterpart characteristics: exon 19 deletion (compared to exon 21 L858R), never-smoker (compared to ever-smoker), and female sex (compared to male sex). The differences in PFS HRs were statistically significant for all three subgroup pairs with or without adjustment for the other two variables, suggesting no significant interplay among them (adjusted P=0.004, P=0.01, P=0.03 for EGFR mutation types, smoking status, and sex, respectively; unadjusted P=0.004, 0.02, P=0.02 for EGFR mutation types, smoking status, and sex, respectively). In the same study, the investigators found no statistically significant association between EGFR mutation type and smoking status (P=0.81) or sex (P=0.81). These findings suggest that the potential mechanism of any PFS advantage in female is independently associated with the EGFR mutation, sex, and smoking status.
ALK and ROS1 inhibitors
Although not as prevalent as EGFR mutations, ALK and ROS1 rearrangements represent targetable genetic alterations present in about 3% and less than 2% of NSCLC patients, respectively (53). Kinase inhibitors used to target ALK rearrangements, such as crizotinib, ceritinib, and lorlatinib, can also be used against ROS1 rearrangements and are included in both ALK-positive and ROS1-positive treatment algorithms of the NCCN guidelines (54). The current literature lacks evidence either supporting or refuting any sex-based differences in patient outcomes with ALK or ROS1 inhibitors, although one meta-analysis reports similar benefits from ALK-TKIs between males and females based on the results from four trials (two on crizotinib vs. chemotherapy and two on ceritinib vs. chemotherapy) (24). Additionally, a few studies provide conflicting results on any sex-based difference in ALK gene alteration (55-57). More studies are needed before a firm conclusion regarding sex differences to ALK and ROS1 inhibitors can be drawn.
Other targeting agents
For other targeted therapy agents, evidence of sex-based differences in drug response is limited due to their relatively recent entry to the drug development scene as well as the lower mutation frequencies of their target genes. Some sex differences have been reported at the molecular level of those clinical actionable genes. Alterations in the MET signaling pathway are able to bypass the inhibition from the EGFR pathway, which is a resistance mechanism leading to disease progression in patients receiving EGFR-TKIs (50,58). Higher c-MET amplification has been detected in samples from lymph nodes of male NSCLC patients (28%) compared to female samples (8%), but this is not reflected in the primary tumor sample (59). Alterations on ErbB2 genes also mediate resistance to EGFR-TKIs, and these mutations are enriched in female NSCLC patients. Out of 224 tumor biopsies, human epidermal growth factor receptor 2 (HER2) mutation was detected in 8 samples, all of which were from female patients (60). In addition, HER2/neu gene expression has been demonstrated as a prognostic factor in female NSCLC patients but not in males (higher HER2/neu expression is associated with worse survival profile) (61). Recently, inhibitors for KRASG12C mutations, such as adagrasib, have been tested in clinical studies. Nearly half of patients with positive KRASG12C mutations showed partial response when treated with adagrasib (62). Although the sex differences in response to KRAS-G12C inhibitor have not been reported, female sex (13.6%) is associated with a higher mutation frequency of KRASG12C than male (10.4%) (63).
Sex differences in responding to immunotherapy in NSCLC
Immune checkpoint blockade
Overview
Since the approval of the first immune checkpoint blockade (ICB) agent, ipilimumab, by the FDA in 2011, immunotherapy has begun to revolutionize the treatment for cancers (64). ICBs are antibodies that can inhibit the interaction of cell surface ligands and modulate the immune response of T cells (65). Anti-cytotoxic T lymphocyte-associated antigen 4 (anti-CTLA4) antibodies such as ipilimumab block the interaction between CTLA4 and CD80/86, which in turn prevents the immune inhibitory effect. Another type of ICB is the anti-programmed cell death protein 1 (anti-PD-1)/anti-PD ligand 1 (anti-PD-L1) antibody. PD-L1 is frequently expressed in immunosuppressive cancer cells, and cancer cells with high expression of PD-L1 exhibit ability to escape the immune surveillance. PD-1/PD-L1 antibodies such as pembrolizumab and nivolumab can block the interaction between PD-1 and PD-L1, thereby reactivating the cytotoxic effect of T cells at the tumor site (65-67).
ICBs, either combined with chemotherapy or as monotherapy agents, have been approved to treat advanced-stage NSCLC without targetable biomarkers (66). However, despite the approval of ICBs in NSCLC, results from phase III trials indicate divergent responses. Randomized controlled trials demonstrate an improved patient survival on pembrolizumab over chemotherapy in treating late-stage NSCLC, while nivolumab is not associated with prolonged PFS compared to chemotherapy (68,69). Such varied response between patients emphasizes the need for effective biomarkers for ICBs. Patient characteristics as well as immune parameters and components have all been investigated in predicting ICBs’ efficacy in NSCLC. Among clinical characteristics, negative predictive values for ICBs’ efficacy have been established in liver and brain metastases (66). Among many molecular features, positive response to ICB treatment has been associated with high tumor mutation burden (TMB), microsatellite instability (MSI), high PD-L1 expression and percentage of infiltrating CD8+ cytotoxic T cells, and low neutrophil-to-lymphocyte ratio (NLR) in NSCLC patients (70,71). In addition, certain signature mutations have been associated with response to ICBs. For example, Kras/Lkb1 (STK11) mutation has been found to drive the resistance to anti-PD-1/PD-L1 therapy in lung adenocarcinoma (65,72). A combination of immune parameters and components have been reported to strongly predict the efficacy of pembrolizumab (73). By categorizing patients based on TMB and expression of signature genes for inflamed T cells, these new biomarkers reflect both tumor antigenicity and tumor microenvironment. Comprehensive overviews of predictive biomarkers for ICB treatment have been reported (65,70).
Female has better response in combination of ICBs with chemotherapy than male
The debates about sex differences in response to ICBs originated from the conflicting findings from several meta-analyses. In a pan-cancer study, Conforti et al. have reported a sex-dependent benefit (P=0.0019) of ICBs in patients with advanced cancers where male patients (HR of OS =0.72, 95% CI: 0.65–0.79) show a better response to ICBs compared to female patients (HR of OS =0.86, 95% CI: 0.79–0.93) (34). This sex difference has not been observed in the subgroup analysis of ICB categories and cancer types. In contrast, Wallis et al. have reported that there is no difference in the ICBs’ efficacy between sexes; in this analysis, male (HR of OS =0.75, 95% CI: 0.69–0.81) and female (HR of OS =0.77, 95% CI: 0.67–0.88) patients benefitted from ICBs equally (35). The main difference between these two large-scale meta-analyses is the selection of published clinical trials. For example, in Wallis's report, four studies that have been included in Confonti's report are removed. These four studies show male advantages in OS over females. In order to avoid the introduction of bias, Ye et al. have conducted an independent meta-analysis by including clinical trial results mentioned in both meta-analyses (74). No significant differences in the efficacy (HR women/men of OS =1.07, 95% CI: 0.95–1.19) of ICBs have been observed between the two sexes. Interestingly, among all cancers included in the meta-analysis, 6 of 11 NSCLC trials show male advantages in OS while one of the NSCLC trial results indicate a significant benefit of the intervention group in OS in female. In this trial, female patients (HR of OS =0.29, 95% CI: 0.19–0.44) responded significantly better to pembrolizumab plus chemotherapy compared to male patients (HR of OS =0.70, 95% CI: 0.50–0.99) (75).
The treatment design of the intervention group seems to significantly impact whether sex difference in response to ICBs is observed in NSCLC patients. A new meta-analysis conducted by Conforti et al. demonstrated a greater benefit from anti-PD-1/PD-L1 ICBs plus chemotherapy in female lung cancer patients than in males (HR men/women of OS =1.56, 95% CI: 1.21–2.01). Furthermore, they found that the sex differential benefits depended on the therapeutic strategy employed. Specifically, female patients saw greater efficacy from combining anti-PD-1/PD-L1 ICBs and chemotherapy compared to male patients (HR men/women of OS is 1.70, 95% CI: 1.16–2.49), whereas male patients tended to benefit more than female patients from anti-PD-1/PD-L1 ICBs alone (HR men/women of OS =0.83, 95% CI: 0.65–1.06) (36). These observations highlight the interaction of treatment setting and sex in the response of anti-PD-1/PD-L1 ICBs in NSCLC. These observations have also been confirmed by another meta-analysis that identifies sex, histology, age, and the type of ICBs as significant predictors of OS for patients who receive combination therapy of anti-PD-1/PD-L1 ICBs and chemotherapy. Female (HR of OS =0.32, 95% CI: 0.23–0.46), non-squamous (HR of OS =0.61, 95% CI: 0.44–0.84) patients who received anti-PD-1 ICBs (HR of OS =0.56, 95% CI: 0.47–0.67) plus chemotherapy tended to have better OS. Significant benefit differences (P<0.001) have been found between females and males (33).
The current clinical guideline suggests the use of anti-PD1/PD-L1 therapy in NSCLC patients with high expression of PD-L1. A Follow-up meta-analysis by Conforti et al. confirmed a male-favored response of anti-PD1/PD-L1 monotherapy verse platinum-based chemotherapy in NSCLC patients with higher expression of PD-L1 in cancer cells (HR men/women of OS =0.71, 95% CI: 0.52–0.98; P=0.04), which suggests a sex heterogeneous response even in patients selected for tumors highly responsive to ICBs (39). These findings suggest possible consideration of sex as a stratification variable when deciding a treatment plan involving ICBs.
Smoking status is associated with the ICBs response in NSCLC. Never smoker tends to have limited benefits from ICBs treatment than ever/current smoker. In our review, the Conforti meta-analyses (39) have included mostly smokers and the Empower-Lung trial (76) was conducted only in ever/current smokers. Both studies found sex differences in ICB responses and neither found smoking history to impact these observations. It is worth noting these conclusions may only be applicable in smokers and that smoking is attributed to a more inflamed tumor microenvironment, which might lead to a greater response of ICBs treatments. Moreover, a superior response to chemotherapy was observed in never-smokers (77). This might be in part explained the better response in females when ICBs are given along with chemotherapy.
Sex differences in ICBs biomarkers
The observed sex differences in patient response to ICBs are multifactorial. Indeed, differences between sexes such as immune system, disease types, genetics, and behavioral aspects, as well as the heterogeneity of treatment setting and dose regimen, complicated the consideration of sex as a determinant of ICBs’ efficacy. This complication highlights the need to understand the underlying biology attributed to the observed sex differences, which in turn will help optimize treatment choice and utilization in NSCLC patients (78).
Currently, there are limited reports on sex differences in the predictive biomarkers of response to ICBs in the context of NSCLC. By using a combination of TMB and T cell inflamed gene expression profile (GEP), a strong predictor of pan-cancer pembrolizumab efficacy has been established (73). High TMB and GEP signature indicate an active immune response in the tumor microenvironment and are associated with better pembrolizumab response. Interestingly, a higher percentage male patients (42%, 81/191) are categorized in the high TMB and GEP group compared to female patients (26%, 29/111) (79). However, this analysis is not representative of NSCLC, since no NSCLC patients were included in this study. In another study, a slight benefit from anti-PD-1/PD-L1 ICBs has been observed in female NSCLC patients, although this was not statistically significant. Moreover, APOBEC (“apolipoprotein B mRNA editing enzyme, catalytic polypeptide-like”), which is associated with the immunotherapy response in NSCLC, shows a female-biased trend. No clear differences have been observed in TMB and PD-L1 expression level (74).
Although sex differences are not observed in biomarkers of ICBs, the predictability of those biomarkers may differ between sexes. A significantly better predictability of TMB in ICB efficacy has been observed in female NSCLC patients compared to males. Such sex differences were also observed in the prognostic power of TMB (80). The expression of PD-L1 is another biomarker of response to ICBs and is currently utilized in the NCCN guideline of NSCLC. However, the prediction power of PD-L1 expression shows no difference between sexes (81).
Methods, such as machine learning models, have been reported to forecast the ICBs response in cancer patients. A random forest model consists of 16 patient characteristics that achieved great sensitivity (pan-cancer area under the curve (AUC): 0.79; NSCLC AUC: 0.82) in predicting ICBs response. Sex is one of the input features but contributes less than others in the model. This might be due to the correlation between sex and other features, such as BMI (82). Therefore, it may be clinically inappropriate to only use a singular parameter to categorize patients. Prospective studies that are carefully designed to investigate the predictive power of each parameter as well as its interactions with other factors in determining responses to ICBs are needed to gain a better understanding of what has been clinically observed.
Sex differences in the molecular features of tumor
Sex differences in the immune features of tumor microenvironment have been comprehensively analyzed, and a disease-dependency has been reported (74). For example, more activated CD4 T cells have been observed in melanomas from male patients, whereas female-enrichment of CD4 T cells have been observed in lung squamous cell carcinoma [TCGA- Lung squamous cell carcinoma (LUSC)]. In NSCLC, more female-enriched immune features (such as T cell inflamed GEP, cytolytic activity, CD4 T cells, and CD8 T cells compositions) have been observed in LUSC but not in LUAD. In another study, Conforti et al. also observed a stronger structured immune response against NSCLC in females, such as a higher abundance of intratumor plasmacytoid and activated dendritic cells, CD4+, and CD8+ T cells. However, immune-suppressive regulators or cells have also been observed in female NSCLC tumor microenvironments (TME), such as cancer-associated fibroblasts (CAFs) and Myeloid-derived suppressor cells (MDSCs), and regulatory T cells (Tregs). Those negative regulators or cells potential lead to the dysfunction infiltrating T cells in TME, therefore, explained the less response of female NSCLC patients when treated with anti-PD1/PD-L1 monotherapy. On the other hand, lower infiltrating immune cells have been noted in the TME, which might be because of the less antigen presentation to the immune system, one of the potential explanations is that the higher degree of hypoxia in the male TME. Tumors characterized with higher glycolysis/OXPHOS ratio had lower expression of genes that related to the antigen-presenting process, including major histocompatibility complex (MHC) molecules (83). These observations indicate a combined effect of sex, cancer biology, and TME in patient response to ICB treatment. The interplay of these elements needs further elucidation.
Sex differences have been observed in the evolution of tumor immunity. Response to ICBs can be determined by the immune recognition of MHC-presented mutant peptides from cancer cells (67). A reduced presentation of immunogenic peptides can cause cancer cells to evade immune surveillance. In a pan-cancer setting, the tumors in female patients tend to have less visible driver mutations that are presented to the immune system than in male patients (84). This potentially is a consequence of a strong immune selection effect in females, in which cancer cells with visible driver mutations are eliminated by the immune system during the early tumorigenesis process.
TP53 is the most frequently mutated gene in NSCLC. A recent report demonstrated sex differences in immune features when patients were stratified by TP53 mutation status (85). The tumor microenvironment of female LUAD patients with wild-type TP53 is enriched with immune signatures of INF-gamma and lymphocyte infiltration as well as the dominant M1 macrophage population in comparison to male patients with wild-type TP53. Such differences are not observed in TP53-mutated LUAD patients. On the other hand, PD-L1 shows higher expression in the mutated TP53 LUAD tumor. However, no significant difference is observed between sexes. These findings correspond with the survival advantage seen in female wild-type TP53 LUAD patients. In contrast, different results are observed in LUSC patients. Mutated TP53 is dominant in this pathological subtype. Immune signatures (such as INF-gamma and lymphocyte infiltration) and better prognosis have been observed in female TP53-mutated LUSC patients compared to males. Although these observations do not necessarily correlate with therapeutic response to ICBs, this work emphasizes the importance of considering sex when stratifying patients using molecular markers and histological subtypes.
A crosstalk between sex chromosomes and TP53 pathway genes has been reported in the pan-cancer setting (86). Non expressed mutations (NEMs) in X chromosomes emerge more frequently in females than in males, and a high incidence of NEMs belongs to the p53-network gene set, such as E3 Ubiquitin Protein Ligase 1 (HUWE1), ATP-dependent helicase ATRX (ATRX). This further indicates a female-biased protective effect that restricts the expression of somatic mutations.
Conclusions
Sex differences of cancers have been well documented in terms of both incidence and mortality through comprehensive epidemiological research (87). However, sex differences in treatment response, which heavily impact the survival outcomes of cancer patients, are reported sparsely. In NSCLC, the critical roles of targeted therapy and ICBs as first/second-line treatments have been well established (88). Despite reported sex-differences in drug response, such as female patients being more likely to benefit than males from EGFR-TKIs, the implementation of sex as a factor to stratify patients prior to treatment is rare in current practice. In this review, we summarized the existing clinical evidence of sex differences in therapeutic response of NSCLC patients to targeted therapy and ICBs. We observed a trend of better survival response from female NSCLC patients with first-generation EGFR-TKIs but no clear sex differences in response to second- or third-generation EGFR-TKIs specifically. Confounding effects from behaviors and ethnicity have also interplayed in the sex differences of response to EGFR-TKIs. In regard to ICBs, conflicting results have been observed among meta-analyses (34,35). Sex differences in response to ICBs are impacted by each patient’s disease-context, clinical characteristics, and treatment options (33). Greater benefits have been observed in female patients when combining ICBs with platinum-doublets, whereas greater benefits have been observed in males when ICBs were given without chemotherapy (36,39). These results indicate that sex interplays with other patient characteristics and that singularly investigating each factor is not the best way to understand the underlying effects of this interplay. More robust evidence is needed to confirm sex as a clinical ICBs efficacy stratification factor/biomarker in treating NSCLC.
Overall, sex differences in therapeutic response have been recognized in clinical settings for NSCLC patients. Although differences in molecular features have been discovered and may explain at least partially the observed sex differences, the evidence only covers a handful of drugs. Careful and comprehensive genetic examination can provide insights on intrinsic biological interpretation for the role of sex in drug response.
Acknowledgments
Funding: This study was supported by NIH/NCI Grants R01CA229618. R.S.H. also received support from NIH/NCI R01CA204856, a research grant from the Avon Foundation for Women and a University of Minnesota (UMN) OACA Faculty Research Development grant. Y.H. received a 3M Fellowship, a UMN Bighley Graduate Fellowship, and a UMN Clinical & Translational Science Institute (CTSI) A-PReP Fellowship.
Footnote
Reporting Checklist: The authors have completed the Narrative Review reporting checklist. Available at https://tlcr.amegroups.com/article/view/10.21037/tlcr-21-1013/rc
Conflicts of Interest: All authors have completed the ICMJE uniform disclosure form (available at https://tlcr.amegroups.com/article/view/10.21037/tlcr-21-1013/coif). The authors have no conflicts of interest to declare.
Ethical Statement: The authors are accountable for all aspects of the work in ensuring that questions related to the accuracy or integrity of any part of the work are appropriately investigated and resolved.
Open Access Statement: This is an Open Access article distributed in accordance with the Creative Commons Attribution-NonCommercial-NoDerivs 4.0 International License (CC BY-NC-ND 4.0), which permits the non-commercial replication and distribution of the article with the strict proviso that no changes or edits are made and the original work is properly cited (including links to both the formal publication through the relevant DOI and the license). See: https://creativecommons.org/licenses/by-nc-nd/4.0/.
References
- Siegel RL, Miller KD, Fuchs HE, et al. Cancer Statistics, 2021. CA Cancer J Clin 2021;71:7-33. [Crossref] [PubMed]
- Duma N, Santana-Davila R, Molina JR. Non–Small Cell Lung Cancer: Epidemiology, Screening, Diagnosis, and Treatment. Mayo Clin Proc 2019;94:1623-40. [Crossref] [PubMed]
- Howlader N, Forjaz G, Mooradian MJ, et al. The Effect of Advances in Lung-Cancer Treatment on Population Mortality. N Engl J Med 2020;383:640-9. [Crossref] [PubMed]
- Khramtsova EA, Davis LK, Stranger BE. The role of sex in the genomics of human complex traits. Nat Rev Genet 2019;20:173-90. [Crossref] [PubMed]
- Haupt S, Caramia F, Klein SL, et al. Sex disparities matter in cancer development and therapy. Nat Rev Cancer 2021;21:393-407. [Crossref] [PubMed]
- Madla CM, Gavins FKH, Merchant HA, et al. Let's talk about sex: Differences in drug therapy in males and females. Adv Drug Deliv Rev. 2021;175:113804. [Crossref] [PubMed]
- Bain C, Feskanich D, Speizer FE, et al. Lung cancer rates in men and women with comparable histories of smoking. J Natl Cancer Inst 2004;96:826-34. [Crossref] [PubMed]
- Devesa SS, Bray F, Vizcaino AP, et al. International lung cancer trends by histologic type: male:female differences diminishing and adenocarcinoma rates rising. Int J Cancer 2005;117:294-9. [Crossref] [PubMed]
- Osann KE, Anton-Culver H, Kurosaki T, et al. Sex differences in lung-cancer risk associated with cigarette smoking. Int J Cancer 1993;54:44-8. [Crossref] [PubMed]
- Lubin JH, Blot WJ. Assessment of lung cancer risk factors by histologic category. J Natl Cancer Inst 1984;73:383-9. [Crossref] [PubMed]
- Midha A, Dearden S, McCormack R. EGFR mutation incidence in non-small-cell lung cancer of adenocarcinoma histology: a systematic review and global map by ethnicity (mutMapII). Am J Cancer Res 2015;5:2892-911. [PubMed]
- Zhang YL, Yuan JQ, Wang KF, et al. The prevalence of EGFR mutation in patients with non-small cell lung cancer: a systematic review and meta-analysis. Oncotarget 2016;7:78985-93. [Crossref] [PubMed]
- Mauvais-Jarvis F, Bairey Merz N, Barnes PJ, et al. Sex and gender: modifiers of health, disease, and medicine. Lancet 2020;396:565-82. [Crossref] [PubMed]
- Geller SE, Koch AR, Roesch P, et al. The More Things Change, the More They Stay the Same: A Study to Evaluate Compliance With Inclusion and Assessment of Women and Minorities in Randomized Controlled Trials. Acad Med 2018;93:630-5. [Crossref] [PubMed]
- Arteaga CL. Overview of epidermal growth factor receptor biology and its role as a therapeutic target in human neoplasia. Semin Oncol 2002;29:3-9. [Crossref] [PubMed]
- Rossi S, Toschi L, Finocchiaro G, et al. Impact of Exon 19 Deletion Subtypes in EGFR-Mutant Metastatic Non–Small-Cell Lung Cancer Treated With First-Line Tyrosine Kinase Inhibitors. Clin Lung Cancer 2019;20:82-7. [Crossref] [PubMed]
- Chan BA, Hughes BG. Targeted therapy for non-small cell lung cancer: current standards and the promise of the future. Transl Lung Cancer Res 2015;4:36-54. [PubMed]
- Siegel-Lakhai WS, Beijnen JH, Schellens JHM. Current Knowledge and Future Directions of the Selective Epidermal Growth Factor Receptor Inhibitors Erlotinib (Tarceva®) and Gefitinib (Iressa®). Oncologist 2005;10:579-89. [Crossref] [PubMed]
- Wirth SM. Afatinib in Non-Small Cell Lung Cancer. J Adv Pract Oncol 2015;6:448-55. [PubMed]
- Wang X, Goldstein D, Crowe PJ, et al. Antitumour effects and mechanisms of action of the panHER inhibitor, dacomitinib, alone and in combination with the STAT3 inhibitor, S3I-201, in human sarcoma cell lines. Int J Oncol 2018;52:2143-54. [Crossref] [PubMed]
- Takeda M, Nakagawa K. First- and Second-Generation EGFR-TKIs Are All Replaced to Osimertinib in Chemo-Naive EGFR Mutation-Positive Non-Small Cell Lung Cancer? Int J Mol Sci 2019;20:146. [Crossref] [PubMed]
- Ettinger DS, Wood DE, Aisner DL, et al. NCCN Guidelines Insights: Non-Small Cell Lung Cancer, Version 2.2021. J Natl Compr Canc Netw 2021;19:254-66. [Crossref] [PubMed]
- Chen X, Liu Y, Røe OD, et al. Gefitinib or erlotinib as maintenance therapy in patients with advanced stage non-small cell lung cancer: a systematic review. PLoS One 2013;8:e59314. [Crossref] [PubMed]
- Pinto JA, Vallejos CS, Raez LE, et al. Gender and outcomes in non-small cell lung cancer: an old prognostic variable comes back for targeted therapy and immunotherapy? ESMO Open 2018;3:e000344. [Crossref] [PubMed]
- Hasegawa Y, Ando M, Maemondo M, et al. The Role of Smoking Status on the Progression-Free Survival of Non-Small Cell Lung Cancer Patients Harboring Activating Epidermal Growth Factor Receptor (EGFR) Mutations Receiving First-Line EGFR Tyrosine Kinase Inhibitor Versus Platinum Doublet Chemot. Oncologist 2015;20:307-15. [Crossref] [PubMed]
- Xiao J, Zhou L, He B, et al. Impact of Sex and Smoking on the Efficacy of EGFR-TKIs in Terms of Overall Survival in Non-small-Cell Lung Cancer: A Meta-Analysis. Front Oncol 2020;10:1531. [Crossref] [PubMed]
- Wheatley-Price P, Blackhall F, Lee SM, et al. The influence of sex and histology on outcomes in non-small-cell lung cancer: a pooled analysis of five randomized trials. Ann Oncol 2010;21:2023-8. [Crossref] [PubMed]
- Wang S, Xing P, Yang K, et al. Efficacy and safety of afatinib in a Chinese population with advanced lung adenocarcinoma with sensitive EGFR mutations. Thorac Cancer 2019;10:1461-8. [Crossref] [PubMed]
- Petrelli F, Borgonovo K, Cabiddu M, et al. Erlotinib as maintenance therapy in patients with advanced non-small cell lung cancer: a pooled analysis of three randomized trials. Anticancer Drugs 2011;22:1010-9. [Crossref] [PubMed]
- Lee CK, Wu YL, Ding PN, et al. Impact of Specific Epidermal Growth Factor Receptor (EGFR) Mutations and Clinical Characteristics on Outcomes After Treatment With EGFR Tyrosine Kinase Inhibitors Versus Chemotherapy in EGFR-Mutant Lung Cancer: A Meta-Analysis. J Clin Oncol 2015;33:1958-65. [Crossref] [PubMed]
- Lee CK, Davies L, Wu YL, et al. Gefitinib or Erlotinib vs Chemotherapy for EGFR Mutation-Positive Lung Cancer: Individual Patient Data Meta-Analysis of Overall Survival. J Natl Cancer Inst 2017; [Crossref] [PubMed]
- Huang L, Huang H, Zhou XP, et al. Osimertinib or EGFR-TKIs/chemotherapy in patients with EGFR-mutated advanced nonsmall cell lung cancer: A meta-analysis. Medicine (Baltimore) 2019;98:e17705. [Crossref] [PubMed]
- Zhou Y, Chen C, Zhang X, et al. Immune-checkpoint inhibitor plus chemotherapy versus conventional chemotherapy for first-line treatment in advanced non-small cell lung carcinoma: a systematic review and meta-analysis. J Immunother Cancer 2018;6:155. [Crossref] [PubMed]
- Conforti F, Pala L, Bagnardi V, et al. Cancer immunotherapy efficacy and patients’ sex: a systematic review and meta-analysis. Lancet Oncol 2018;19:737-46. [Crossref] [PubMed]
- Wallis CJD, Butaney M, Satkunasivam R, et al. Association of Patient Sex With Efficacy of Immune Checkpoint Inhibitors and Overall Survival in Advanced Cancers: A Systematic Review and Meta-analysis. JAMA Oncol 2019;5:529-36. [Crossref] [PubMed]
- Conforti F, Pala L, Bagnardi V, et al. Sex-Based Heterogeneity in Response to Lung Cancer Immunotherapy: A Systematic Review and Meta-Analysis. J Natl Cancer Inst 2019;111:772-81. [Crossref] [PubMed]
- Khan M, Lin J, Liao G, et al. Comparative analysis of immune checkpoint inhibitors and chemotherapy in the treatment of advanced non-small cell lung cancer: A meta-analysis of randomized controlled trials. Medicine (Baltimore) 2018;97:e11936. [Crossref] [PubMed]
- Lee CK, Man J, Lord S, et al. Clinical and Molecular Characteristics Associated With Survival Among Patients Treated With Checkpoint Inhibitors for Advanced Non-Small Cell Lung Carcinoma: A Systematic Review and Meta-analysis. JAMA Oncol 2018;4:210-6. [Crossref] [PubMed]
- Conforti F, Pala L, Pagan E, et al. Sex-based differences in response to anti-PD-1 or PD-L1 treatment in patients with non-small-cell lung cancer expressing high PD-L1 levels. A systematic review and meta-analysis of randomized clinical trials. ESMO Open 2021;6:100251. [Crossref] [PubMed]
- Yuan Y, Liu L, Chen H, et al. Comprehensive Characterization of Molecular Differences in Cancer between Male and Female Patients. Cancer Cell 2016;29:711-22. [Crossref] [PubMed]
- Chen J, Yang H, Teo ASM, et al. Genomic landscape of lung adenocarcinoma in East Asians. Nat Genet 2020;52:177-86. [Crossref] [PubMed]
- Sugio K, Uramoto H, Ono K, et al. Mutations within the tyrosine kinase domain of EGFR gene specifically occur in lung adenocarcinoma patients with a low exposure of tobacco smoking. Br J Cancer 2006;94:896-903. [Crossref] [PubMed]
- Tanaka T, Matsuoka M, Sutani A, et al. Frequency of and variables associated with the EGFR mutation and its subtypes. Int J Cancer 2010;126:651-5. [Crossref] [PubMed]
- Hsiao SH, Lin SE, Chou YT, et al. Histological subtype and smoking status, but not gender, are associated with epidermal growth factor receptor mutations in non-small-cell lung cancer. Mol Clin Oncol 2014;2:252-8. [Crossref] [PubMed]
- Ko R, Kenmotsu H, Serizawa M, et al. Frequency of EGFR T790M mutation and multimutational profiles of rebiopsy samples from non-small cell lung cancer developing acquired resistance to EGFR tyrosine kinase inhibitors in Japanese patients. BMC Cancer 2016;16:864. [Crossref] [PubMed]
- Huang YH, Hsu KH, Tseng JS, et al. The Association of Acquired T790M Mutation with Clinical Characteristics after Resistance to First-Line Epidermal Growth Factor Receptor Tyrosine Kinase Inhibitor in Lung Adenocarcinoma. Cancer Res Treat 2018;50:1294-303. [Crossref] [PubMed]
- Zhou Y, Ma Y, Shi H, et al. Epidermal growth factor receptor T790M mutations in non-small cell lung cancer (NSCLC) of Yunnan in southwestern China. Sci Rep 2018;8:15426. [Crossref] [PubMed]
- Li H, Wang J, Zhang G, et al. Detection of plasma T790M mutation after the first generation EGFR-TKI resistance of non-small cell lung cancer in the real world. J Thorac Dis 2020;12:550-7. [Crossref] [PubMed]
- Lee Y, Lee GK, Hwang JA, et al. Clinical likelihood of sporadic primary EGFR T790M Mutation in EGFR-mutant Lung Cancer. Clin Lung Cancer 2015;16:46-50. [Crossref] [PubMed]
- Passaro A, Janne PA, Mok T, et al. Overcoming therapy resistance in EGFR-mutant lung cancer. Nat Cancer 2021;2:377-91. [Crossref] [PubMed]
- Tomizawa Y, Iijima H, Sunaga N, et al. Clinicopathologic significance of the mutations of the epidermal growth factor receptor gene in patients with non-small cell lung cancer. Clin Cancer Res 2005;11:6816-22. [Crossref] [PubMed]
- Hsieh RK, Lim KH, Kuo HT, et al. Female sex and bronchioloalveolar pathologic subtype predict EGFR mutations in non-small cell lung cancer. Chest 2005;128:317-21. [Crossref] [PubMed]
- Bergethon K, Shaw AT, Ou SH, et al. ROS1 rearrangements define a unique molecular class of lung cancers. J Clin Oncol 2012;30:863-70. [Crossref] [PubMed]
- Herbst RS, Morgensztern D, Boshoff C. The biology and management of non-small cell lung cancer. Nature 2018;553:446-54. [Crossref] [PubMed]
- Fallet V, Cadranel J, Doubre H, et al. Prospective screening for ALK: clinical features and outcome according to ALK status. Eur J Cancer 2014;50:1239-46. [Crossref] [PubMed]
- Cabillic F, Gros A, Dugay F, et al. Parallel FISH and immunohistochemical studies of ALK status in 3244 non-small-cell lung cancers reveal major discordances. J Thorac Oncol 2014;9:295-306. [Crossref] [PubMed]
- Incharoen P, Reungwetwattana T, Saowapa S, et al. ALK-rearranged pulmonary adenocarcinoma in Thai Patients: From diagnosis to treatment efficacy. World J Surg Oncol 2016;14:139. [Crossref] [PubMed]
- Aldea M, Andre F, Marabelle A, et al. Overcoming Resistance to Tumor-Targeted and Immune-Targeted Therapies. Cancer Discov 2021;11:874-99. [Crossref] [PubMed]
- Xu CW, Wang WX, Wu MJ, et al. Comparison of the c-MET gene amplification between primary tumor and metastatic lymph nodes in non-small cell lung cancer. Thorac Cancer 2017;8:417-22. [Crossref] [PubMed]
- Li C, Sun Y, Fang R, et al. Lung adenocarcinomas with HER2-activating mutations are associated with distinct clinical features and HER2/EGFR copy number gains. J Thorac Oncol 2012;7:85-9. [Crossref] [PubMed]
- Vallböhmer D, Brabender J, Yang DY, et al. Sex differences in the predictive power of the molecular prognostic factor HER2/neu in patients with non-small-cell lung cancer. Clin Lung Cancer 2006;7:332-7. [Crossref] [PubMed]
- Riely GJ, Ou SHI, Rybkin I, et al. Activity and Preliminary Pharmacodynamic (PD) Analysis of Adagrasib (MRTX849) in Patients (Pts) With Advanced Non–Small- Cell Lung Cancer (NSCLC) Harboring KRASG12C Mutation. J Thorac Oncol 2021;16:S751-2. [Crossref]
- Nassar AH, Adib E, Kwiatkowski DJ. Distribution of KRAS G12C Somatic Mutations across Race, Sex, and Cancer Type. N Engl J Med 2021;384:185-7. [Crossref] [PubMed]
- Sadozai H, Gruber T, Hunger RE, et al. Recent Successes and Future Directions in Immunotherapy of Cutaneous Melanoma. Front Immunol 2017;8:1617. [Crossref] [PubMed]
- Havel JJ, Chowell D, Chan TA. The evolving landscape of biomarkers for checkpoint inhibitor immunotherapy. Nat Rev Cancer 2019;19:133-50. [Crossref] [PubMed]
- Passaro A, Attili I, Morganti S, et al. Clinical features affecting survival in metastatic NSCLC treated with immunotherapy: A critical review of published data. Cancer Treat Rev 2020;89:102085. [Crossref] [PubMed]
- Ribas A, Wolchok JD. Cancer immunotherapy using checkpoint blockade. Science 2018;359:1350-5. [Crossref] [PubMed]
- Carbone DP, Reck M, Paz-Ares L, et al. First-Line Nivolumab in Stage IV or Recurrent Non–Small-Cell Lung Cancer. N Engl J Med 2017;376:2415-26. [Crossref] [PubMed]
- Reck M, Rodríguez-Abreu D, Robinson AG, et al. Pembrolizumab versus Chemotherapy for PD-L1-Positive Non-Small-Cell Lung Cancer. N Engl J Med 2016;375:1823-33. [Crossref] [PubMed]
- Lesterhuis WJ, Bosco A, Millward MJ, et al. Dynamic versus static biomarkers in cancer immune checkpoint blockade: unravelling complexity. Nat Rev Drug Discov 2017;16:264-72. [Crossref] [PubMed]
- Valero C, Lee M, Hoen D, et al. Pretreatment neutrophil-to-lymphocyte ratio and mutational burden as biomarkers of tumor response to immune checkpoint inhibitors. Nat Commun 2021;12:729. [Crossref] [PubMed]
- Hellmann MD, Nathanson T, Rizvi H, et al. Genomic Features of Response to Combination Immunotherapy in Patients with Advanced Non-Small-Cell Lung Cancer. Cancer Cell 2018;33:843-852.e4. [Crossref] [PubMed]
- Cristescu R, Mogg R, Ayers M, et al. Pan-tumor genomic biomarkers for PD-1 checkpoint blockade-based immunotherapy. Science 2018;362:eaar3593. [Crossref] [PubMed]
- Ye Y, Jing Y, Li L, et al. Sex-associated molecular differences for cancer immunotherapy. Nat Commun 2020;11:1779. [Crossref] [PubMed]
- Gandhi L, Rodríguez-Abreu D, Gadgeel S, et al. Pembrolizumab plus Chemotherapy in Metastatic Non-Small-Cell Lung Cancer. N Engl J Med 2018;378:2078-92. [Crossref] [PubMed]
- Sezer A, Kilickap S, Gümüş M, et al. Cemiplimab monotherapy for first-line treatment of advanced non-small-cell lung cancer with PD-L1 of at least 50%: a multicentre, open-label, global, phase 3, randomised, controlled trial. Lancet 2021;397:592-604. [Crossref] [PubMed]
- Tsao AS, Liu D, Lee JJ, et al. Smoking affects treatment outcome in patients with advanced nonsmall cell lung cancer. Cancer 2006;106:2428-36. [Crossref] [PubMed]
- Klein SL, Flanagan KL. Sex differences in immune responses. Nat Rev Immunol 2016;16:626-38. [Crossref] [PubMed]
- Conforti F, Pala L, Bagnardi V, et al. Sex-based differences of the tumor mutational burden and T-cell inflammation of the tumor microenvironment. Ann Oncol 2019;30:653-5. [Crossref] [PubMed]
- Wang S, Zhang J, He Z, et al. The predictive power of tumor mutational burden in lung cancer immunotherapy response is influenced by patients' sex. Int J Cancer 2019;145:2840-9. [Crossref] [PubMed]
- Wang S, Cowley LA, Liu XS. Sex Differences in Cancer Immunotherapy Efficacy, Biomarkers, and Therapeutic Strategy. Molecules 2019;24:3214. [Crossref] [PubMed]
- Chowell D, Yoo SK, Valero C, et al. Improved prediction of immune checkpoint blockade efficacy across multiple cancer types. Nat Biotechnol 2022;40:499-506. [Crossref] [PubMed]
- Conforti F, Pala L, Pagan E, et al. Sex-Based Dimorphism of Anticancer Immune Response and Molecular Mechanisms of Immune Evasion. Clin Cancer Res 2021;27:4311-24. [Crossref] [PubMed]
- Castro A, Pyke RM, Zhang X, et al. Strength of immune selection in tumors varies with sex and age. Nat Commun 2020;11:4128. [Crossref] [PubMed]
- Freudenstein D, Litchfield C, Caramia F, et al. TP53 Status, Patient Sex, and the Immune Response as Determinants of Lung Cancer Patient Survival. Cancers (Basel) 2020;12:1535. [Crossref] [PubMed]
- Haupt S, Caramia F, Herschtal A, et al. Identification of cancer sex-disparity in the functional integrity of p53 and its X chromosome network. Nat Commun 2019;10:5385. [Crossref] [PubMed]
- Zhu Y, Shao X, Wang X, et al. Sex disparities in cancer. Cancer Lett 2019;466:35-8. [Crossref] [PubMed]
- Clinical N, Guidelines P, Guidelines N. Non-Small Cell Lung. 2021. Available online:
10.6004/jnccn.2021.0013 10.6004/jnccn.2021.0013