BRAF mutant non-small cell lung cancer and treatment with BRAF inhibitors
Introduction
The mitogen-activated protein kinase (MAPK) kinase (MEKK)/extracellular signal-regulated kinase (ERK) cascades are membrane-to-nucleus signaling modules highly conserved from yeast to vertebrates and involved in multiple physiological processes. The RAS-RAF-MEK-ERK kinase pathway mediates cellular responses to growth signals (1). BRAF is a member of the RAF family of serine/threonine protein kinases. There are three RAF isoforms in humans: ARAF, BRAF, and CRAF (also called Raf-1). These serine/threonine protein kinases are components of a conserved signaling pathway downstream of the membrane-bound small G protein RAS, which is activated by growth factors, hormones, and cytokines (2). RAS stimulates and recruits RAF proteins to the cell membrane where they are activated (3). Active BRAF then activates a second protein kinase called MEK, which in turn activates a third protein kinase called ERK. Once activated, ERK can translocate into the nucleus where phosphorylates transcription factors, thereby regulating their activity (Figure 1). ERK regulates gene expression, cytoskeletal rearrangements, and metabolism to coordinate responses to extracellular signals and regulate proliferation, differentiation, angiogenesis, senescence, and apoptosis.
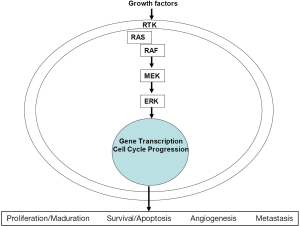
The three RAF proteins are not equal in their ability to activate MEK. A-RAF is a poor MEK activator. BRAF has been identified as the major MEK activator, and displays higher affinity for MEK1 and MEK2 than RAF-1 (4). The structure of RAF proteins consists of an amino terminus that contains the regulatory domain, an activation loop, and a carboxyl terminus that contains the kinase domain. CR1 and CR2 in the N terminus are regulatory, whereas CR3 at the C terminus encompases the kinase domain. RAS recruits cytosolic RAF to the plasma membrane for activation by phosphorylation of two amino acids within the activation segment of the kinase domain (5). The differential regulation of the RAF isoforms lies in phosphorylation of a motif called the negative-charge regulatory region (N region). CRAF and ARAF must be phosphorylated for maximal activation. Unlike ARAF and CRAF, the N region of BRAF carries a constitutive negative charge and N region phosphorylation is not required, so BRAF is primed for activation (6). This constitutive phosphorylation is the cause of the higher basal activity of B-RAF.
All RAF isoforms activate MEK1, whereas only BRAF and CRAF activate MEK2. Although both ARAF and CRAF are capable of activating other signaling elements independent of MAPK pathway activation, such as nuclear factor-kappa B (NF-κB), Rb, and Bcl-2, MEK1 and MEK2 are the only known substrates for BRAF (7-10).
BRAF mutations in lung cancer
The RAS-RAF-MEK-ERK pathway can be constitutively activated by alterations in specific proteins, including BRAF. BRAF mutations have been identified in a wide range of cancers including 50% of malignant melanomas, 45% of papillary thyroid cancer, 10% of colorectal cancers, and also in ovarian, breast, and lung cancers (11-13). Somatic activating BRAF mutations were first described by Davies et al. in 2002 (11). Genomic DNA from 545 cancer cell lines and the corresponding matched lymphoblastoid cell lines from the same individuals were screened for sequence variants through the coding exons and intron-exon junctions of the BRAF gene. They reported an incidence of 8% across all cancers (43/545) and 3% in lung cancer (all adenocarcinomas) (4/131) (Table 1). All BRAF somatic mutations found in the cancer lines were in exons 11 and 15. RAS and BRAF mutations usually occur in the same cancer types, but these mutations are found in a mutually exclusive fashion (11).
Over 40 different missense mutations in B-RAF, involving 24 different codons, have been identified in human cancer. The majority of BRAF mutations localize to the kinase domain and increase the kinase activity of BRAF toward MEK. Most mutations are extremely rare, accounting for 0.1-2% of all cases. However, the most common is a thymidine to adenosine transversion at nucleotide T1799A at exon 15, which results in a valine to glutamate substitution at codon 600 (V600E) (11). It accounts for most (over 90%) of the mutations in melanoma and thyroid cancer and for a high proportion of those in colorectal cancer, but is comparatively rare in non small cell lung cancer (NSCLC). This mutation appears to mimic regulatory phosphorylation and increases BRAF activity approximately 500-fold compared to wild-type (14). In melanoma, colon and breast cancer cells harbouring V600E mutation, cyclin D1 expression, and cell cycle progression are MEK-dependent (15).
Reinforcing its role as an oncogene, lung-specific expression of V600E BRAF in mice leads to the development of lung cancers with bronchioalveolar carcinoma features similar to those observed in patients. Deinduction of transgene expression led to dramatic tumor regression, paralleled by dramatic dephosphorylation of ERK1/2, implying a dependency of BRAF-mutant lung tumors on the MAPK pathway. The growth of these tumors was dependent on persistent oncogene expression, suggesting that mutant BRAF may also be necessary for maintenance (16). But many of the non-V600E mutations show only intermediate and low kinase activity, so their classification as driver mutations remains in doubt (14). Probably V600E mutants overcome the need for a RAS-dependent step by mimicking phosphorylation, whereas the less common BRAF mutants still require interaction with RAS to become phosphorylated and activated (11).
To study the biology of BRAF mutation in NSCLC, Pratilas et al. (17) screened a panel of 87 lung cancer cell lines for exons 11 and 15 BRAF mutations. They identified five cell lines with known hotspot mutations within the BRAF kinase domain, all derived from patients with a histologic diagnosis of adenocarcinoma, one with V600E and four with non-V600E mutations. They observed that NSCLC with BRAF mutations were selectively sensitive to MEK inhibition compared with cell lines harboring mutations in epidermal growth factor receptor (EGFR), KRAS, or ALK and ROS kinase fusions. MEK inhibition in V600E BRAF NSCLC cells led to induction of apoptosis, comparable with that seen with EGFR kinase inhibition in EGFR mutant NSCLC models. Despite high basal ERK phosphorylation, EGFR mutant cells were resistant to MEK inhibition, and BRAF mutant cell lines were resistant to EGFR inhibition. Additionally, they studied the frequency of BRAF mutations in tumors of 916 patients with NSCLC, sequencing the exons 11 and 15 of BRAF. They identified 17 mutant cases or 1.9%, most in adenocarcinoma histology (88%), female gender (64.7%), and former or current smokers (70.6%). BRAF V600E was present in 11/17 tumors (65%), that is, 1.2% of the total number of NSCLC tumors, all with adenocarcinoma histology (Table 1).
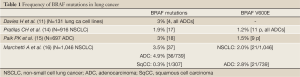
Full Table
An institutional lung cancer mutation analysis program performed at Memorial Sloan-Kettering Cancer Center confirmed the incidence (18). Six-hundred and ninety-seven patients with lung adenocarcinoma underwent molecular testing for EGFR, KRAS, and BRAF mutations and ALK rearrangements. BRAF mutations were present in 18 patients (3%) (Table 1), EGFR mutations in 165 patients (24%), KRAS mutations in 169 patients (25%), and ALK translocations in 44 patients (6%). No patient with a BRAF mutation had a concomitant mutation in EGFR or KRAS or a translocation in ALK. The BRAF mutations identified were V600E (50%), G469A (39%), and D594G (11%). In contrast to patients with EGFR mutations and ALK rearrangements who were mostly never smokers, all patients with BRAF mutations were current or former smokers (P<0.001). Patients with KRAS mutations were also predominantly smokers. Female gender was predominant in patients with BRAF mutations (11/18, 61%), including the V600E variant (7/9, 77.8%). There were no significant differences in overall survival for advanced-stage patients with BRAF mutations compared with other driver mutations. The median overall survival of advanced-stage patients with BRAF mutations was not reached. In comparison, the median overall survival of patients with EGFR mutations was 37 months (P=0.73), with KRAS mutations was 18 months (P=0.12), and with ALK rearrangements was not reached (P=0.64).
A retrospective series of 1,046 patients with surgically resected NSCLC, comprising 739 adenocarcinomas and 307 squamous cell carcinomas (SqCC), was investigated for RAF mutations in Italy (19). Tumor DNA was subjected to high-resolution melting analysis, and the alterations detected were confirmed by sequencing. BRAF mutations were detected in 37 tumors (3.5%) (Table 1). BRAF mutations were present in 36 adenocarcinomas (4.9%) and one SqCC (0.3%; P=0.001): 56.8% (21/37) were V600E, all in adenocarcinoma subtype, and 43.2% (16/37) were non-V600E. The incidence of V600E mutations in adenocarcinomas was 2.8% (21/739). V600E mutations were significantly more prevalent in females (16 of 187 patients; 8.6%) than in males (5 of 552 patients; 0.9%), as indicated by multivariate logistic regression analysis [Hazard Ratio (HR), 11.29; P<0.001]. The proportion of females in the V600E group was 76% (16/21). Also, V600E mutations were significantly more prevalent in never smokers (10 of 197 patients; 5.1%) than in smokers or former smokers (11 of 542 patients; 2%; P=0.04). Other clinicopathologic parameters, including age, tumor size, nodal status, and tumor stage, were not significantly associated with V600E BRAF mutations. V600E tumors showed an aggressive histotype characterized by micropapillary features in 80% of patients. Patients with V600E mutations had shorter median disease-free survival (DFS) and overall survival (OS) rates than patients without V600E mutations (15.2 vs. 52.1 months; P<0.001, and 29.3 vs. 72.4 months; P<0.001, respectively). All non-V600E mutations detected in adenocarcinomas (15/739; 2%) were found in smokers (P=0.015) and showed micropapillary features in 12% of patients. No differences in DFS and OS were observed between patients with and without non-V600E mutations (42.8 vs. 43.2 months; P=0.84, and 56.4 vs. 65.1 months; P=0.42, respectively). Multivariate analysis confirmed that pathologic stage and V600E mutations were the only independent and significant factors to predict DFS (HR, 2.54; P<0.001 and HR, 2.19; P=0.011, respectively) and OS (HR, 2.92; P<0.001 and HR, 2.18; P=0.014, respectively). Patients were also analyzed for KRAS and EGFR mutations. KRAS mutations were observed in 203 patients (27%) and EGFR mutations in 86 (12%). All tumors with BRAF mutations were found to be negative for KRAS mutations. BRAF V600E and EGFR were concomitantly mutated in two tumors.
NSCLC treatment with BRAF inhibitors
Most cancer cells harboring a V600E BRAF mutation display a critical dependence on the activity of this oncogene for their growth and survival, and are extremely sensitive to selective BRAF and MEK inhibitors. The analysis of V600E mutation may identify a subset of lung cancers sensitive to BRAF inhibition, resulting in significantly improved outcomes for these patients.
New selective inhibitors of mutant BRAF have generated considerable interest. Vemurafenib (PLX4032) and dabrafenib (GSK2118436) are the most studied V600E BRAF inhibitors.
Vemurafenib is a potent inhibitor of BRAF with the V600E mutation, with no antitumor effects against cells with wild-type BRAF (20-22). A phase 1 trial established the recommended phase 2 dose to be 960 mg twice daily and showed tumor responses (23). A phase 2 trial in patients with previously treated BRAF V600-mutant metastatic melanoma to investigate the efficacy of vemurafenib showed a response rate of 53% (6% complete response and 47% partial response), with a median duration of response of 6.7 months and a median OS of 15.9 months (24). A phase 3 randomized trial comparing vemurafenib with dacarbazine in patients with previously untreated, metastatic melanoma with the BRAF V600E mutation reported an OS at 6 months of 84% in the vemurafenib group and 64% in the dacarbazine group (25). Response rates were 48% for vemurafenib and 5% for dacarbazine. Vemurafenib was associated with a relative reduction of 63% in the risk of death and 74% in the risk of tumor progression, as compared with dacarbazine (P<0.001 for both comparisons). The estimated median progression-free survival (PFS) was 5.3 months in the vemurafenib group and 1.6 months in the dacarbazine group. Common adverse events (AE) related to vemurafenib were arthralgia, rash, fatigue, alopecia, keratoacanthoma or squamous-cell carcinoma, photosensitivity, nausea, and diarrhea; 38% of patients required dose modification because of toxic effects. A case report of a male patient with BRAF V600E lung adenocarcinoma showed a good clinical and metabolic response when treated with vemurafenib (26).
Dabrafenib (GSK2118436) is a reversible, potent and selective inhibitor of BRAF V600E kinase activity consistent with adenosine triphosphate-competitive inhibition. The maximum tolerated dose was not reached in phase I study (27). The dose of 150 mg oral twice a day was selected based on the pharmacokinetics and the effects of dabrafenib on a molecular biomarker target (tumor pERK inhibition), FDG-PET metabolic uptake, disease assessment as measured by response per RECIST criteria at first restaging, and the safety profile. Dose administration of 200 mg twice daily did not provide higher exposure (Cmax and AUC) relative to 150 mg twice daily by day 15. Activity of dabrafenib was shown in V600E BRAF-mutant (Val600Glu) and in those with Val600Lys BRAF-mutant metastatic melanoma. Whereas the proportion of patients who responded was lower in patients with Val600Lys than in those with Val600Glu BRAF-mutant melanoma, PFS was equivalent at the recommended phase 2 dose.
In a multicentre, open-label, phase 2 trial (28), dabrafenib demonstrated clinical evidence of activity in subjects with BRAF V600E mutation positive melanoma with brain metastases who were local treatment naive (cohort A) as well as those who previously received local therapy (cohort B). Overall intracranial response rate (OIRR) and median duration of OIRR were 39.2% and 5.0 months, and 30.8% and 7.0 months, respectively. Two complete responses were recorded in cohort A. PFS and OS were 4 months and 8 months in both groups. Of the 172 patients enrolled, 33 (19%) had Val600Lys BRAF-mutant disease. In this group, fewer patients achieved an OIRR than did those with Val600Glu BRAF-mutant melanoma: one of 15 patients (6.7%) in cohort A, as did four of 18 (22.2%) in cohort B. Median OS was also shorter for patients with Val600Lys BRAF-mutant metastatic melanoma. Cutaneous squamous-cell carcinoma or keratoacanthoma occurred in 6% of patients in this study. All lesions were excised. BRAF-inhibitor induced cutaneous squamous-cell carcinoma occurs because a paradoxical RAF inhibitor-mediated activation of the RAS signaling pathway in BRAF wild-type cells (29).
Furthermore, dabrafenib showed a response rate of 59% in a phase 2 trial assessing the efficacy in patients with BRAF mutation positive metastatic melanoma (30).
In phase III study of dabrafenib versus dacarbazine in patients with unresectable or metastatic BRAF V600E mutation positive melanoma, treatment with dabrafenib resulted in significant improvement in PFS, response rate, and duration of response over dacarbazine (31). The estimated median PFS for the dabrafenib group was 5.1 months and 2.7 months for the dacarbazine group, with a HR of 0.30 (95% CI, 0.18-0.51; P<0.0001). The preliminary overall survival HR was 0.61 (95% CI, 0.25-1.48) in favour of dabrafenib. Response rate was 50% in the dabrafenib group (3% complete response and 47% partial response) and 6% in the dacarbazine group. Most patients randomly assigned to dabrafenib experienced some degree of reduction in target lesion size. 28 patients randomly assigned to dacarbazine had crossed over to dabrafenib. The majority of all AE reported in melanoma patients treated with dabrafenib were grade 1 or 2. The most common AEs of any grade were hyperkeratosis, headache, pyrexia, arthralgia, skin papilloma, palmar-plantar erythrodysaesthesia syndrome, fatigue, and nausea. Serious AEs reported were cutaneous squamous-cell carcinoma (8%), basal cell carcinoma, pyrexia, malignant melanoma, hypokalemia, hyponatremia, and neutropenia. Dose reduction of dabrafenib was needed in 52 (28%) patients, and five (3%) patients discontinued drug because of AEs.
Taking into account all this data, prospective genotyping of NSCLC patients for BRAF V600E mutation is justified, and could also have the benefit of assessing the efficacy of BRAF selective inhibitors. A phase 2, international, multicenter, non-randomized, open-label trial is being conducted in 69 centers from USA, Europe and Asia.
Forty patients will be included; 24 have been recruited. Patients with confirmed stage IV V600E BRAF mutant NSCLC who have relapsed or progressed on one or more platinum-based chemotherapy regimens will be screened for eligibility. Eligible patients will receive dabrafenib 150 mg twice daily and continue treatment until disease progression, unacceptable adverse events or death. Subjects still benefiting from dabrafenib at the time of study completion may have the option to enter the protocol BRF114144, an open-label, rollover study of dabrafenib as monotherapy or in combination with other anti-cancer treatments. The primary objective is to assess the overall response rate, and secondary objective is to assess PFS, duration of response and OS. Additional objectives are to characterize the safety and tolerability of dabrafenib as a single agent, to characterize the pharmacokinetics of dabrafenib, and to explore the molecular mechanisms of sensitivity and resistance to dabrafenib.
This study is an opportunity to assess the incidence of V600E BRAF mutation in NSCLC patients, and to evaluate the efficacy of dabrafenib in this selected population.
Although the response of the disease to agents directed to a molecular target is usually very good in a high proportion of patients, eventually cancer cells will develop acquired resistance with continuous dosing.
The frequency with which the downstream MAP kinase pathway remains active in tumours resistant to BRAF inhibition has led to a phase 1 and 2 study of dabrafenib combined with the MEK inhibitor trametinib (GSK1120212), for patients with BRAF-mutant metastatic melanoma. In the phase 2 part of the trial, patients were randomly assigned to receive combination therapy with dabrafenib and trametinib or dabrafenib monotherapy. Median PFS in the combination group was 9.4 months, as compared with 5.8 months in the monotherapy group (HR for progression or death, 0.39; 95% CI, 0.25 to 0.62; P<0.001). The rate of complete or partial response with combination therapy was 76%, as compared with 54% with monotherapy (P=0.03) (32).
The mechanism of resistance to BRAF inhibitors seems to involve reactivation of the MAPK pathway upstream of MEK, including the up-regulation of bypass pathways mediated by cancer Osaka thyroid kinase (COT) (33), development of de novo NRAS or MEK mutations (34,35), and dimerization or variant splicing of mutant BRAF V600 (36). MAPK-independent signaling through receptor tyrosine kinases, such as platelet-derived growth factor receptor beta (34), insulin-like growth factor 1 receptor (37), and hepatocyte growth factor receptor (38).
Future strategies and combination of targeted therapies, such of the combination of dabrafenib and trametinib, could overcome or prevent resistance to BRAF inhibitors.
Conclusions
Although lung cancer is still a devastating disease, and the major cause of death for cancer, the treatment outcome of patients with metastatic NSCLC has been improved by the application of new knowledge about cancer biology and molecular alterations in the origin of the tumor. BRAF mutations are frequent in melanoma, and targeted treatments directed against the BRAF V600E mutation have changed the treatment of patients with BRAF-mutant melanoma. It is expected that in lung cancer these drugs will provide high response rates and durable clinical benefit as do other targeted therapies against driver mutations. A phase 2 trial to evaluate the antitumor efficacy of dabrafenib in lung adenocarcinomas harboring the V600E BRAF mutation is currently underway, and could establish this mutation as a predictive marker in a subset of lung cancer patients. Rationally designed combination regimens to overcome resistance mechanisms might prolong the benefit from BRAF inhibitor treatment.
Acknowledgements
Disclosure: The authors declare no conflict of interest.
References
- Peyssonnaux C, Eychène A. The Raf/MEK/ERK pathway: new concepts of activation. Biol Cell 2001;93:53-62. [PubMed]
- Robinson MJ, Cobb MH. Mitogen-activated protein kinase pathways. Curr Opin Cell Biol 1997;9:180-6. [PubMed]
- Garnett MJ, Marais R. Guilty as charged: B-RAF is a human oncogene. Cancer Cell 2004;6:313-9. [PubMed]
- Papin C, Denouel-Galy A, Laugier D, et al. Modulation of kinase activity and oncogenic properties by alternative splicing reveals a novel regulatory mechanism for B-Raf. J Biol Chem 1998;273:24939-47. [PubMed]
- Zhang BH, Guan KL. Activation of B-Raf kinase requires phosphorylation of the conserved residues Thr598 and Ser601. EMBO J 2000;19:5429-39. [PubMed]
- Mason CS, Springer CJ, Cooper RG, et al. Serine and tyrosine phosphorylations cooperate in Raf-1, but not B-Raf activation. EMBO J 1999;18:2137-48. [PubMed]
- Kolch W. Meaningful relationships: the regulation of the Ras/Raf/MEK/ERK pathway by protein interactions. Biochem J 2000;351:289-305. [PubMed]
- Baumann B, Weber CK, Troppmair J, et al. Raf induces NF-kappaB by membrane shuttle kinase MEKK1, a signaling pathway critical for transformation. Proc Natl Acad Sci U S A 2000;97:4615-20. [PubMed]
- Wang S, Ghosh RN, Chellappan SP. Raf-1 physically interacts with Rb and regulates its function: a link between mitogenic signaling and cell cycle regulation. Mol Cell Biol 1998;18:7487-98. [PubMed]
- Wang HG, Miyashita T, Takayama S, et al. Apoptosis regulation by interaction of Bcl-2 protein and Raf-1 kinase. Oncogene 1994;9:2751-6. [PubMed]
- Davies H, Bignell GR, Cox C, et al. Mutations of the BRAF gene in human cancer. Nature 2002;417:949-54. [PubMed]
- Dhomen N, Marais R. BRAF signaling and targeted therapies in melanoma. Hematol Oncol Clin North Am 2009;23:529-45. [PubMed]
- Xing M. BRAF mutation in thyroid cancer. Endocr Relat Cancer 2005;12:245-62. [PubMed]
- Wan PT, Garnett MJ, Roe SM, et al. Mechanism of activation of the RAF-ERK signaling pathway by oncogenic mutations of B-RAF. Cell 2004;116:855-67. [PubMed]
- Solit DB, Garraway LA, Pratilas CA, et al. BRAF mutation predicts sensitivity to MEK inhibition. Nature 2006;439:358-62. [PubMed]
- Ji H, Wang Z, Perera SA, et al. Mutations in BRAF and KRAS converge on activation of the mitogen-activated protein kinase pathway in lung cancer mouse models. Cancer Res 2007;67:4933-9. [PubMed]
- Pratilas CA, Hanrahan AJ, Halilovic E, et al. Genetic predictors of MEK dependence in non-small cell lung cancer. Cancer Res 2008;68:9375-83. [PubMed]
- Paik PK, Arcila ME, Fara M, et al. Clinical characteristics of patients with lung adenocarcinomas harboring BRAF mutations. J Clin Oncol 2011;29:2046-51. [PubMed]
- Marchetti A, Felicioni L, Malatesta S, et al. Clinical features and outcome of patients with non-small-cell lung cancer harboring BRAF mutations. J Clin Oncol 2011;29:3574-9. [PubMed]
- Bollag G, Hirth P, Tsai J, et al. Clinical efficacy of a RAF inhibitor needs broad target blockade in BRAF-mutant melanoma. Nature 2010;467:596-9. [PubMed]
- Tsai J, Lee JT, Wang W, et al. Discovery of a selective inhibitor of oncogenic B-Raf kinase with potent antimelanoma activity. Proc Natl Acad Sci U S A 2008;105:3041-6. [PubMed]
- Joseph EW, Pratilas CA, Poulikakos PI, et al. The RAF inhibitor PLX4032 inhibits ERK signaling and tumor cell proliferation in a V600E BRAF-selective manner. Proc Natl Acad Sci U S A 2010;107:14903-8. [PubMed]
- Flaherty KT, Puzanov I, Kim KB, et al. Inhibition of mutated, activated BRAF in metastatic melanoma. N Engl J Med 2010;363:809-19. [PubMed]
- Sosman JA, Kim KB, Schuchter L, et al. Survival in BRAF V600-mutant advanced melanoma treated with vemurafenib. N Engl J Med 2012;366:707-14. [PubMed]
- Chapman PB, Hauschild A, Robert C, et al. Improved survival with vemurafenib in melanoma with BRAF V600E mutation. N Engl J Med 2011;364:2507-16. [PubMed]
- Gautschi O, Pauli C, Strobel K, et al. A patient with BRAF V600E lung adenocarcinoma responding to vemurafenib. J Thorac Oncol 2012;7:e23-4. [PubMed]
- Falchook GS, Long GV, Kurzrock R, et al. Dabrafenib in patients with melanoma, untreated brain metastases, and other solid tumours: a phase 1 dose-escalation trial. Lancet 2012;379:1893-901. [PubMed]
- Long GV, Trefzer U, Davies MA, et al. Dabrafenib in patients with Val600Glu or Val600Lys BRAF-mutant melanoma metastatic to the brain (BREAK-MB): a multicentre, open-label, phase 2 trial. Lancet Oncol 2012;13:1087-95. [PubMed]
- Poulikakos PI, Zhang C, Bollag G, et al. RAF inhibitors transactivate RAF dimers and ERK signalling in cells with wild-type BRAF. Nature 2010;464:427-30. [PubMed]
- Trefzer U, Minor D, Ribas A, et al. BREAK-2: a phase IIA trial of the selective BRAF kinase inhibitor GSK2118436 in patients with BRAF mutation-positive (V600E/K) metastatic melanoma. Pigment Cell Res 2011;24:abstr LBA1-1.
- Hauschild A, Grob JJ, Demidov LV, et al. Dabrafenib in BRAF-mutated metastatic melanoma: a multicentre, open-label, phase 3 randomised controlled trial. Lancet 2012;380:358-65. [PubMed]
- Flaherty KT, Infante JR, Daud A, et al. Combined BRAF and MEK inhibition in melanoma with BRAF V600 mutations. N Engl J Med 2012;367:1694-703. [PubMed]
- Johannessen CM, Boehm JS, Kim SY, et al. COT drives resistance to RAF inhibition through MAP kinase pathway reactivation. Nature 2010;468:968-72. [PubMed]
- Nazarian R, Shi H, Wang Q, et al. Melanomas acquire resistance to B-RAF(V600E) inhibition by RTK or N-RAS upregulation. Nature 2010;468:973-7. [PubMed]
- Emery CM, Vijayendran KG, Zipser MC, et al. MEK1 mutations confer resistance to MEK and B-RAF inhibition. Proc Natl Acad Sci U S A 2009;106:20411-6. [PubMed]
- Poulikakos PI, Persaud Y, Janakiraman M, et al. RAF inhibitor resistance is mediated by dimerization of aberrantly spliced BRAF(V600E). Nature 2011;480:387-90. [PubMed]
- Villanueva J, Vultur A, Lee JT, et al. Acquired resistance to BRAF inhibitors mediated by a RAF kinase switch in melanoma can be overcome by cotargeting MEK and IGF-1R/PI3K. Cancer Cell 2010;18:683-95. [PubMed]
- Straussman R, Morikawa T, Shee K, et al. Tumour micro-environment elicits innate resistance to RAF inhibitors through HGF secretion. Nature 2012;487:500-4. [PubMed]