Alternative to surgery in early stage NSCLC—interventional radiologic approaches
Introduction
The standard of care for operable patients with early stage non-small cell lung cancer (NSCLC) is lobectomy with lymph node evaluation (1,2). However, a significant number of patients with early stage NSCLC are not candidates for lobectomy due to diminished pulmonary function and other comorbidities (3-5). For non-surgical candidates, stereotactic body radiation therapy (SBRT) or image-guided percutaneous thermal ablation are attractive options.
For more than a decade, thermal ablation has been an effective, safe, repeatable, and relatively low-cost technique in the treatment of various solid tumors, including in the liver, kidney, adrenal gland, breast and bone (6). In 2000, Dupuy et al. first reported the use of radiofrequency ablation (RFA) in the treatment of lung tumors (7). Since then, RFA has been the most widely used form of thermal ablation in the lung, including in the treatment of medically inoperable or high-risk early stage NSCLC (8-14). In recent years, microwave ablation and cryoablation have also been applied with increasing frequency (15-19).
Percutaneous thermal ablation offers may advantages including its minimally invasive nature, its ability to preserve normal lung parenchyma with minimal effect on pulmonary function, and the ability to perform these procedure under moderate sedation or even local anesthesia (16,19). In fact, most procedures are performed in a single outpatient session and can even be done at the same time as a biopsy (20). Furthermore, percutaneous thermal ablation allows for repeated treatment sessions, which may improve survival in patients who have failed primary treatment (21). Repeated surgery, on the other hand, is often not feasible secondary to either technical difficulty or limited residual pulmonary reserves. SBRT is similarly limited in regards to retreatment of local tumor recurrence secondary to limitations in maximal tolerated radiation dosages to the lung for fear of radiation pneumonitis. Another limitation of some types of SBRT is the need for multiple gold fiducial markers, which are placed percutaneously or bronchoscopically. Percutaneous placement of fiducial markers is associated with higher complication rates than percutaneous thermal ablation (22).
In this article, we will review the three different thermal ablative modalities, including patient selection, technique, treatment outcomes, complications, and imaging follow-up. A brief discussion on state of the art techniques such as irreversible electroporation (IRE) and catheter directed therapies will also be included.
Patient selection and pre-procedural evaluation
In the treatment of early stage NSCLC, image-guided percutaneous thermal ablation is indicated for patients who are not surgical candidates due to cardiopulmonary comorbidities such as severe chronic obstructive pulmonary disease (7,12,18,19,23). Ablative techniques may be performed in patients who have limited pulmonary function. Pulmonary function is generally not significantly changed after thermal ablation. In fact, even in post-pneumonectomy patients with a single lung, RF ablation may be safely performed with preservation of pulmonary function (24,25).
Evaluation of patients prior to thermal ablation entails taking a history and physical examination with attention to bleeding diathesis and medications such as anticoagulants and antiplatelet agents. Medical comorbidities need to be assessed to determine the safety of administering moderate conscious sedation or general anesthesia. Patients should also be screened for the presence of cardiac devices because the energy from RFA may potentially interfere with pacemakers or defibrillators; these patients should have their treatment sessions coordinated with a cardiac electrophysiologist (26). However, this cardiac risk is obviated by bipolar microwave ablation systems as well as cryoablation devices.
Recent imaging, such as computed tomography and/or fluorodeoxyglucose positron emission tomography, are important for assessing tumor size and proximity to neurovascular structures as well as for selecting the type, number, and trajectory of ablation probes (27). An inherent limitation of non-surgical therapy is the inability to systematically assess for nodal disease. In one study, among patients with clinical stage I NSCLC, 13.8% of patients were upstaged to N1 disease on final surgical pathology and an additional 3.5% upstaged to N2 disease (28). The presence of nodal or extra-thoracic disease is generally a contraindication to thermal ablation unless the goal of the treatment is palliative.
Prior to the procedure, the potential side effects are explained to the patient including possible post-ablation syndrome, in which an inflammatory response may result in fever, malaise, and anorexia which may persist for several days (29). Patients may also experience post-procedural mild to moderate pain which is usually controlled with analgesics.
Techniques
RFA
RFA uses electromagnetic energy of a specific radiofrequency range, generally 375-500 kHz, to achieve controlled thermal destruction of cells and tissues (30). In RFA, an active electrode is placed into the tumor under image-guidance. A grounding electrode is placed on the opposite side of the chest or thigh. When the two electrodes are connected to an RF generator, a voltage gradient is produced. This voltage gradient results in an oscillating electric field that induces electrons to collide with the molecules closest to the applicator, which produces frictional heat (31). Tissue heating to a temperature greater than 60 degrees Celsius leads to immediate cell death secondary to coagulation necrosis (32).
In RFA of the lung, there are several obstacles that limit effective thermal ablation of tumor. First, pulmonary vessels and airways act as a “heat sink” to dissipate energy away from the adjacent normal lung parenchyma; this “heat sink” effect limits the size of the ablation margin surrounding the tumor (33,34). Second, there is inherent high-impedance in inflated lung due to its low water content, which limits the therapeutic ablative volume of ablation (35). Third, a fundamental limitation of RFA is its inability to heat charred or desiccated tissue (34,36).
Two popular RF devices for the treatment of pulmonary tumors are Starburst Radiofrequency Ablation System (Angiodynamics, Latham, NY) and Cool-tip (Covidien, Boulder, CO). The Starburst device uses a deployable array RF electrode via a 14- to 17-gauge needle. The Cool-tip device uses a cluster electrode that is perfused with cold saline or water pumped internally; this mechanism is designed to distribute tissue heating to reduce charring (37).
Microwave ablation
Microwave ablation uses electromagnetic energy at a much higher frequency range [generally 900-2,450 MHz (38)] compared to RFA and creates a larger zone of coagulation necrosis (18,39). Unlike RFA, microwave energy penetration does not occur by means of an electric current and therefore is thought not limited by the lower electrical conductivity of inflated lung, charred tissue, or desiccated tissue (18,38,40). Furthermore, no grounding pads are used in the microwave ablation. Microwave ablation utilizes rapidly alternating electric fields which cause polar water molecules to spin rapidly. These spinning water molecules then transfer their kinetic energy to the surrounding tissues resulting in hyperthermia (41).
Compared to RFA, microwave ablation can achieve larger ablative zones more quickly with less heat sink effect. In microwave ablation, a single probe may be used for tumors less than 3 cm. Two to three probes are generally used for tumors greater than 3 cm to produce a larger area of thermocoagulation. A thermocouple may be placed separately to measure the intratumoral temperature. Six microwave ablation devices are available for use. The 2,450-MHz generators are Amica (Hospital Service, Rome, Italy), Acculis MTA (Microsulis, Hamsphire, UK), Certus 140 (Neuwave, Madison, WI). The 915-MHz generators are Avecure (Medwaves, San Diego, CA), Evident (Covidien, Mansfield, MA), MicrothermX (BSD Medical, Salt Lake City, UT). The microwave antennae are straight applicators with active tips measuring 0.6 to 4.0 cm in length. The proximal portion of the antennae is cooled with room-temperature fluid or carbon dioxide to minimize damage of skin and tissues (30).
Cryoablation
Percutaneous cryoablation uses pressurized argon gas to achieve temperatures as low as –140 degrees Celsius based on the Joule-Thomson principle. At temperatures less than –40 degrees Celsius, cryogenic tissue destruction occurs due to protein denaturation, cell rupture from osmotic water shifts across cell membranes, as well as microvascular thrombosis-induced ischemia (42).
A freeze-thaw-freeze cycle is used for each cryoprobe to achieve thermal coagulation while minimizing air leak and bleeding (43). The thaw portion of the cycle is performed using helium and the cryoprobe is allowed to reach approximately 20 degrees Celsius. An example of a cryoablation protocol would consist of a 10-minute freeze of the tumor, followed by an 8-minute thaw, and then a 10-minute freeze, followed by an active or passive thaw.
Two cryoablation devices are available: Cryocare (Endocare, Irvine, CA) and Presice (Galil Medical, Arden Mills, MN). A cryoprobe measures 1.5 to 2.4 mm in diameter. One to 15 cryoprobes may be placed at a time with each probe achieving thermocoagulation after a single freeze-thaw-freeze cycle.
A comparison of the aforementioned thermal ablation modalities is summarized in Table 1.
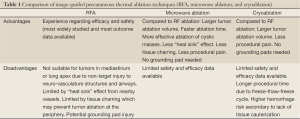
Full Table
Irreversible electroporation
IRE is the newest of the percutaneous ablation techniques with only a few reports of IRE use in human subjects. IRE uses very short high-voltage electrical pulses to create permanent nanopores in tumor cell membranes to induce apoptosis and cell death (44-46). IRE is largely non-thermal and spares the surrounding extracellular matrix. Thus, the theoretical advantage of IRE is that tumor ablation occurs while sparing non-target injury to adjacent airways, blood vessels, and nerves (47). Therefore, IRE may potentially be used to treat pulmonary tumors near the hilum, mediastinum, and chest wall (48).
Preprocedural planning for IRE is essential for two reasons. Patients require general anesthesia and complete neuromuscular blockade to prevent generalized muscle contractions. Second, ECG-gated delivery of IRE is required in the chest to prevent cardiac arrhythmias. There is currently one IRE device approved by the FDA—Nanoknife (Angio Dynamics, Latham, NY). Nanoknife has already been used to ablate tumors in the lung, liver, kidney, prostate, and pancreas. Nanoknife electrodes are monopolar with a retractable sheath which allows for adjustable active length from 1 to 4 cm. Up to six electrodes may be used simultaneously for tumor ablation.
Outcomes in early stage disease
The literature on pulmonary thermal ablation in early stage disease is heterogeneous due to the diversity of study groups (mixture of primary and secondary lung tumors) and variations in follow-up lengths as well as reporting standards. Furthermore, the vast majority of the studies were performed retrospectively at single institutions (Table 2).
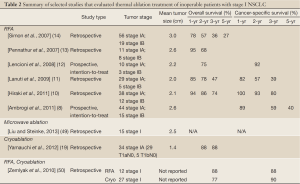
Full Table
Ablation
Among the thermal ablation techniques, RFA has been the most widely used in the treatment of early stage NSCLC. The retrospective study by Simon et al. (14) is the largest to date and included 75 patients with stage I NSCLC who underwent percutaneous CT-guided RF ablation. There were 56 patients with stage IA disease and 19 patients with stage IB disease. The mean tumor diameter was 3.0 cm. The overall 1-, 2-, 3-, 4-, and 5-year survival rates, respectively, for stage I NSCLC were 78%, 57%, 36%, 27%, and 27%. The median survival was 29 months. Local tumor progression-free rates were as follows: 1 year, 83%; 2 years, 64%; 3 years, 57%; 4 years, 47%; and 5 years, 47% for tumors 3 cm or smaller. Tumor size was a statistically significant predictor of local tumor progression in this study: median time to progression was 12 months for tumors >3 cm and 45 months for tumors <3 cm (14). It is worthwhile noting that the Charlson comorbidity index predicted patient outcome in cases of inoperable NSCLC (i.e., much better survival in patients with less comorbidities) (51).
The RAPTURE study by Lencioni et al. (12) was the first prospective, intention-to-treat clinical trial for RFA that reported the outcome of 106 patients with 183 lung tumors, among which only 13 patients had stage I NSCLC (stage IA, n=10; stage IB, n=3). The mean tumor size was 2.2 cm for all patients with NSCLC in this study. The patients with stage I disease had a 2-year overall survival of 75% and a 2-year cancer-specific survival of 92%.
The second prospective, intention-to-treat clinical trial by Ambriogi et al. (8) for RFA was of 57 inoperable patients with stage I NSCLC (stage IA tumor, n=44; stage IB tumor, n=15). The mean tumor size was 2.6 cm. Cancer-specific actuarial survivals were 89%, 59%, and 40% at 1, 3, and 5 years, respectively. The median overall survival was 33.4 months and the cancer-specific survival was 41.4 months.
In a retrospective study by Hiraki et al. (10) of 50 nonsurgical patients with stage I NSCLC (stage IA, n=38; stage IB, n=12) who were treated with RFA, the mean tumor size was 2.1 cm. After 37 months of follow-up, the local progression rate was 31%. The overall survival was 94%, 86%, and 74% at 1, 2, and 3 years, respectively. The cancer-specific survival was 100%, 93%, and 80% at 1, 2, and 3 years, respectively.
In a retrospective study by Lanuti et al. (11) of 31 patients with medically inoperable stage I NSCLC had 34 tumors treated with RFA (stage IA, n=29; stage IB, n=5), the mean size of the treated tumors was 2.0 cm. The overall survivals at 1, 2 and 3 years were 85%, 78% and 47%, respectively. Disease-free survivals at 1, 2 and 3 years were 82%, 57% and 39%, respectively. Local progression-free survivals at 1, 2, and 3 years were 71%, 58%, and 58%. The local failure rate was 32% after a median follow-up of 17 months.
Pennathur et al. (13) reported the outcome of 19 high-risk patients with stage I NSCLC (stage IA, n=11; stage IB, n=8) who underwent RFA. The mean tumor size was 2.6 cm. Overall survivals were 95% and 68% at 1 and 2 years, respectively.
Microwave ablation
The first study of microwave ablation in the lung was performed by Feng et al. (15) but no separate subset analysis of early stage NSCLC was performed. The largest study of microwave ablation was done by Wolf et al. (18) which retrospectively examined the recurrence and survival outcome of 50 patients, among which 27 patients had NSCLC and the remainder had small cell lung cancer or metastatic disease. While, the NSCLC stage was not specified, the overall mean tumor size was 3.5 cm. No subset analysis was done for patients with NSCLC versus other malignancies. These limitations limit this study’s ability to assess the efficacy of microwave ablation in the early stage subgroup (18). While several other studies exist such as those by Belfiore et al. (52) and Lu et al. (53), these studies are similarly limited by either a lack of subgroup analysis or no reports of outcome data.
The only study focused on early stage NSCLC is a preliminary retrospective review by Liu et al. (49) of 15 patients with medically inoperable stage I NSCLC who were treated with CT-guided percutaneous microwave ablation. The mean tumor size was 2.5 cm (range, 0.8-4.0 cm). Local progression was 31% after 1-year follow-up; however, 80% of the local progression was observed in pleural-based tumors that were larger than 3.0 cm. No survival data was reported for this study.
Cryoablation
A study by Yamauchi et al. (19) retrospectively reviewed 20 patients with medically inoperable stage IA NSCLC who had 34 tumors treated with CT-guided cryoblation under local anesthesia. Twenty-nine tumors were T1aN0 and 5 tumors were T1bN0. The mean tumor size was 1.4 cm (range, 0.5-3.0 cm) and 12 tumors were subsolid on CT imaging. The 2- and 3-year overall survivals were 88% and 88%, respectively. One patient died of lung cancer progression at 68 months. Two patients died of acute exacerbations of idiopathic pulmonary fibrosis which were not considered to be directly related to the cryoablation, at 12 and 18 months, respectively. After a median follow-up of 23 months, the local control was 97%. Only one patient, who had a 1.6 cm squamous cell carcinoma, had local tumor progression at 8 months post-treatment; furthermore, in this patient, the local recurrence was re-treated with cryoablation and there was no evidence of further local recurrence. There was no significant change in the pulmonary function tests before and after cryoablation. The excellent survival data and local control for this study may partially be attributed to the small tumor size and the significant number of subsolid lesions.
Wang et al. (17) also performed a retrospective study reviewing the outcome for cryoablation of 234 lung tumors but no specific data was reported for patients with early stage NSCLC.
The retrospective study by Zemlyak et al. (50) included 64 patients, among whom 25 underwent sublobar resection, 12 RFA, and 27 cryoablation. The mean tumor size was not reported in this study. However, the RFA group included patients with “larger lesions (≥3 cm)” while the cryoablation group was performed in patients with “lesions <3 cm”. The 3-year overall survival was 87.1%, 87.5%, and 77% for sublobar resection, RFA, and cryoablation, respectively. The 3-year cancer-specific survival was 90.6%, 87.5%, and 90.2%. This study was limited by the small number of patients and selection bias.
Irreversible electroporation
There is currently very limited data of the use of IRE in human lung. The majority of the outcome data is derived from animal models (47,48). The theoretical advantage of IRE is preservation of underlying lung architecture and surrounding neurovascular structures, which may permit the use of IRE in ablating tumors in the mediastinum and lung apex. Another theoretical advantage is overcoming “heat sink” effect. Thomson et al. (54) performed IRE in 38 patients, among whom 3 patients had advanced lung cancer. All three patients had inadequate treatment response.
Complications
Image-guided percutaneous thermal ablation of lung cancer is generally safe and well-tolerated by most patients even with those with limited cardiopulmonary reserve. Most complications after percutaneous ablation are minor and treated conservatively or with minimal intervention (Table 3). There are, however, rare but serious complications such as massive pulmonary hemorrhage, bronchopleural fistula, and pulmonary artery pseudoaneurysm. Overall, procedural-related death is rare with the mortality rate reported to be 0.4% for RFA (55).
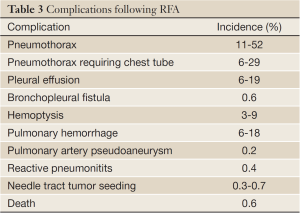
Full Table
The most common complication after percutaneous lung ablation is pneumothorax. Pneumothorax occurs in 11% to 52% of cases, although only 6% to 29% of patients require chest tube placement (21,56-58). Rarely, there may be a bronchopleural fistula, which occurs in 0.6% of patients and is thought to be related to aggressive treatment (59). Pleural effusion is also a relatively common complication reported in 6% to 19% of cases and may be the result of non-target thermal injury to the pleura (21,57,60).
Hemoptysis and pulmonary hemorrhage are not uncommon after thermal ablation with reported incidences being 3-9% (57,61,62) and 6-18% (63,64), respectively, after RFA. Pulmonary hemorrhage and hemoptysis is more common after cryoablation than after RF or microwave ablation (16,43). Cryoablation has no cautery effect that is inherent in RFA or microwave ablation both of which use extreme heat; furthermore, cryoablation results in damage to microcirculation during the thaw cycle. Pulmonary hemorrhage is usually self-limiting and treated conservatively, although there are case reports of uncontrollable hemorrhage leading to death (23,63-65). Pulmonary artery pseudoaneurysm is a rare but life-threatening complication that occurred in 0.2% in a series of RF ablations (66). Two reports of a pulmonary artery pseudoaneurysm after RFA were both successfully treated using transcatheter coil embolization (67,68).
Non-target thermal damage to peripheral nerves may occur depending on the location of the tumor. If the tumor is in the lung apex, thermal ablation may cause injury to the caudal brachial plexus (69). Phrenic nerve injury is another potential complication if ablation is performed in close proximity (<1 cm) of the phrenic nerve (70). A thorough knowledge of the course of these nerves may reduce the rate of these non-target thermal injuries.
Pneumonitis after RFA is a rare but potentially lethal complication. In one series of RFA in the lung, there were two deaths attributed to interstitial pneumonia; both patients received radiotherapy prior to thermal ablation (60). In another series of patients, bronchiolitis obliterans organizing pneumonia-like reactive pneumonitits occurred after RF ablation in 0.4% of patients (71).
A very rare but potentially life-threatening complication following any percutaneous needle-based procedure, including lung biopsy and thermal ablation, is systemic air embolism. There have been only two reported cases of systemic air embolism after RFA in the lung, both of which were nonfatal (72,73). The treatment for systemic air embolism is hyperbaric oxygen therapy.
Another rare complication is tumor seeding in the needle tract with reported incidence of 0.3% to 0.7% (74,75).
Follow-up imaging
Imaging is a critical component of thermal ablation because, unlike surgery, there is no tissue sample available for histopathologic evaluation of the ablative margin. Computed tomography (CT) and/or fluorodeoxyglucose positron emission tomography (FDG-PET) are used to ensure complete ablation and to evaluate for treatment response. There is no consensus on which imaging modality or imaging time interval that most accurately detects treatment success or failure or recurrent disease. However, follow-up imaging protocols after thermal ablation generally entail obtaining CT and/or FDG-PET one month post-ablation and then every three months thereafter (76,77).
After RFA, immediate post-procedural CT images demonstrate central consolidation (coagulation necrosis) surrounded by concentric rings of groundglass opacity (edema, inflammation, hemorrhage) (78,79). As the peripheral groundglass opacity overestimates the area of true coagulation necrosis by 4.1 mm (78), it is recommended that the ablation extend at least 5 mm (18,78,80) beyond the tumor. In one study (81), an ablation area at least four times larger than the pre-ablation tumor was predictive of complete ablation.
Within the first week of RFA, contrast-enhanced CT imaging may show a peripheral thin (<5 mm) rim of enhancement, which reflects benign reactive hyperemia (76). FDG-PET is not useful in the immediate post-ablation setting as there is non-specificity in its findings given the background of expected inflammatory reaction (82).
CT imaging 1-month post-RF ablation demonstrates a consolidated lesion that is larger than the pre-ablation tumor. Cavitation can develop in up to 25% of cases; cavitation is thought to occur as the sequelae of physiologic drainage of necrotic tissue (79,81,83). Two to six months post-RFA, CT imaging shows no change or increased size of the ablation cavity in comparison to the pre-ablation tumor. Because of this expected treatment response, Response Evaluation Criteria in Solid Tumors (RECIST) criteria is not ideal for evaluating changes after thermal ablation.
Beyond 6 months post-RFA, the ablation zone is expected to be smaller in size than the index tumor (84). Tumor recurrence or progression should be suspected if there is increased size of the ablation zone and/or central or nodular enhancement after 6 months (14,80). FDG uptake peaks at approximately 2 weeks post-RF ablation. Increased or new metabolic uptake on FDG-PET imaging at 2 months is suspicious for disease recurrence (85).
After microwave ablation, immediate post-procedural CT shows groundglass/consolidative opacity centrally (coagulation necrosis) with surrounding groundglass opacities (edema, hemorrhage, inflammation) penetrated by well-demarcated probe tracts (Figure 1) (18). The ablation zone increases in size for up to 6 months after treatment due to thermal changes in the adjacent lung parenchyma. After 6 months, the ablation zone should decrease in size and be replaced by consolidation. Cavitation within the ablation zone has been associated with decreased cancer-specific mortality (18), possibly due to cavitary changes occurring more often when there is more complete tumor destruction with thermocoagulation of local tissue blood supply (30). Similar to RFA, central or nodular enhancement on CT as well as increased or new metabolic uptake on FDG-PET imaging is suspicious for treatment failure.
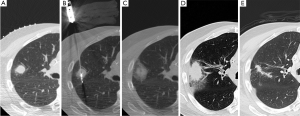
Following the thaw cycle, the cryoablation zone is seen as a low attenuation central area (coagulation necrosis) surrounded by a concentric ring of groundglass opacity (hemorrhage and edema) (43,86). The “ice ball” seen on peri-procedural images overestimates the area of coagulation necrosis by 4 to 5 mm (87-89). After the first month post-ablation, the peripheral groundglass opacity resolves as the central zone of coagulation necrosis becomes well-marginated and consolidative. Cavitation may occur within this central area of necrosis (17). On immediate post-procedural contrast-enhanced CT, there may sometimes be peripheral or internal enhancement in the cryoablation zone that should resolve within 1 to 2 months (86). PET is not performed immediately post-cryoablation due to its nonspecificity in the background of reactive inflammation (Figure 2).
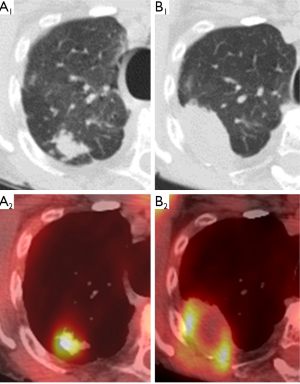
At 1 month post-cryoablation, the ablation zone should be decreasing in size. Growth in the ablation zone during the first two months post-ablation is suspicious for tumor recurrence or progression (86). In contrast to RFA or microwave ablative zones, the cryoablation zone generally involutes earlier and faster which would theoretically allow for earlier detection of treatment failure (17). FDG-PET imaging demonstrating new or increasing uptake after 2 months post-ablation is suggestive of tumor recurrence, whereas the absence of FDG uptake coupled with lesion resolution indicates adequate treatment response (Figure 3).
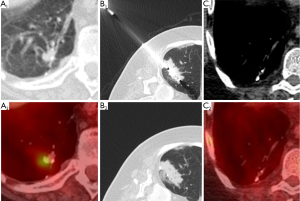
Palliative therapies
Image-guided thermal ablation is also useful in the palliative treatment of lung cancer through local control of advanced or metastatic disease. For example, painful bone metastases may be effectively palliated with RFA or cryoablation (90-93). Currently, there is a multicenter, prospective, single arm study (ECLIPSE trial) that is evaluating the safety and efficacy of cryoablation to patients with pulmonary metastatic disease.
Other palliative interventional radiologic procedures include endovascular stenting for superior vena cava syndrome and bronchial artery embolization for hemoptysis associated with lung cancer (94). Trans-arterial embolization may also be performed for preoperative purposes to decrease blood loss (95,96).
Emerging therapies
Less established in treatment of lung tumors but widely used in treatment of tumors in solid organs such as the liver, transvascular therapy is being reexamined as a potential primary or adjuvant therapy. Several approaches are being investigated, including transpulmonary chemoembolization and image-guided percutaneous- or intra-arterial delivery of therapeutic nanoparticles.
In transpulmonary chemoembolization, the tumor-feeding pulmonary arteries are selectively catheterized after which a mixture of cytotoxic and embolic agents are administered locally. Vogl et al. (96-99) used a femoral vein puncture to access the tumor-supplying pulmonary artery and then infused a combination of lipiodol, mitomycin C, and microspheres to treat metastatic and primary lung tumors. In a study of 17 patients with unresectable primary lung tumors (97), transpulmonary chemoembolization was performed for symptomatic palliation. No major complications occurred with 35% of patients experiencing local progression after a mean follow-up of 11.3 months.
In the future, image-guided minimally invasive procedures will play a role in the delivery of a variety of nanotherapeutics via percutaneous or intravascular methods (100-102). The direct intra-tumoral delivery of therapeutic nanoparticles minimizes systemic toxicity while maximizing local efficacy of tumor destruction. For example, in a new investigational method called “magnetic” chemotherapy, chemotherapeutics are tagged with magnetic nanoparticles. After infusion of the magnetic nanotherapeutics into the vascular supply of the tumor, an external rare earth magnet is placed over the tumor. The resultant magnetic attraction directs the therapeutic particles out of the vessel and into the tumor.
Conclusions
Interventional radiologists play a key role in the image-guided thermal ablation of lung malignancies. RFA has been the most widely used form of thermal ablation in the lung. RFA for inoperable early stage NSCLC compares favorably to SBRT in regards to overall patient survival (8,10-14,19,20,49,50,103-108). However, RFA has worse local control rates than SBRT although this does not appear to affect overall survival as many patients can easily undergo retreatment (20,109). As a more powerful form of thermal ablation, microwave ablation may potentially provide superior local control due to its larger zone of active heating and its ability to achieve higher intratumoral temperatures. There is still limited safety and efficacy data on the use of microwave ablation in early stage NSCLC although its use is growing. The preliminary data on cryoblation is promising, with one study of medically inoperable early stage NSCLC demonstrating a 3-year overall survival of 88% and a local control rate of 97% (19).
In a recent consensus statement by the American College of Chest Physicians and Society of Thoracic Surgeons regarding inoperable early stage NSCLC, image-guided thermal ablation was considered a treatment option only if the patient was not a candidate for SBRT (110). This statement is not surprising given that interventional radiology was not involved in the consensus and there is a paucity of multi-institutional clinical trials for thermal ablation. However, there is currently a multicenter pilot trial of inoperable patients with stage IA NSCLC treated with RFA, which is funded by the National Cancer Institute and performed through the American College of Surgeons Oncology Group (ACOSOG Z4033) (111). Although the results are not yet published, this RFA cohort was recently compared to sublobar resection and SBRT cohorts from other completed multi-center trials, and the survival was similar (despite the RFA cohort being older and sicker) (20).
Further studies need to done to determine which patients would benefit most from image-guided thermal ablation. Future research dictating the tumor location, size, and histology that is most suitable for ablation will help patients to achieve the best outcome.
Acknowledgements
Disclosure: The authors declare no conflict of interest.
References
- Ginsberg RJ, Rubinstein LV. Randomized trial of lobectomy versus limited resection for T1 N0 non-small cell lung cancer. Lung Cancer Study Group. Ann Thorac Surg 1995;60:615-22; discussion 622-3. [PubMed]
- El-Sherif A, Gooding WE, Santos R, et al. Outcomes of sublobar resection versus lobectomy for stage I non-small cell lung cancer: a 13-year analysis. Ann Thorac Surg 2006;82:408-15; discussion 415-6. [PubMed]
- Mery CM, Pappas AN, Bueno R, et al. Similar long-term survival of elderly patients with non-small cell lung cancer treated with lobectomy or wedge resection within the surveillance, epidemiology, and end results database. Chest 2005;128:237-45. [PubMed]
- Raz DJ, Zell JA, Ou SH, et al. Natural history of stage I non-small cell lung cancer: implications for early detection. Chest 2007;132:193-9. [PubMed]
- Bach PB, Cramer LD, Warren JL, et al. Racial differences in the treatment of early-stage lung cancer. N Engl J Med 1999;341:1198-205. [PubMed]
- Dupuy DE, Goldberg SN. Image-guided radiofrequency tumor ablation: challenges and opportunities--part II. J Vasc Interv Radiol 2001;12:1135-48. [PubMed]
- Dupuy DE, Zagoria RJ, Akerley W, et al. Percutaneous radiofrequency ablation of malignancies in the lung. AJR Am J Roentgenol 2000;174:57-9. [PubMed]
- Ambrogi MC, Fanucchi O, Cioni R, et al. Long-term results of radiofrequency ablation treatment of stage I non-small cell lung cancer: a prospective intention-to-treat study. J Thorac Oncol 2011;6:2044-51. [PubMed]
- Hiraki T, Gobara H, Iishi T, et al. Percutaneous radiofrequency ablation for clinical stage I non-small cell lung cancer: results in 20 nonsurgical candidates. J Thorac Cardiovasc Surg 2007;134:1306-12. [PubMed]
- Hiraki T, Gobara H, Mimura H, et al. Percutaneous radiofrequency ablation of clinical stage I non-small cell lung cancer. J Thorac Cardiovasc Surg 2011;142:24-30. [PubMed]
- Lanuti M, Sharma A, Digumarthy SR, et al. Radiofrequency ablation for treatment of medically inoperable stage I non-small cell lung cancer. J Thorac Cardiovasc Surg 2009;137:160-6. [PubMed]
- Lencioni R, Crocetti L, Cioni R, et al. Response to radiofrequency ablation of pulmonary tumours: a prospective, intention-to-treat, multicentre clinical trial (the RAPTURE study). Lancet Oncol 2008;9:621-8. [PubMed]
- Pennathur A, Luketich JD, Abbas G, et al. Radiofrequency ablation for the treatment of stage I non-small cell lung cancer in high-risk patients. J Thorac Cardiovasc Surg 2007;134:857-64. [PubMed]
- Simon CJ, Dupuy DE, DiPetrillo TA, et al. Pulmonary radiofrequency ablation: long-term safety and efficacy in 153 patients. Radiology 2007;243:268-75. [PubMed]
- Feng W, Liu W, Li C, et al. Percutaneous microwave coagulation therapy for lung cancer. Zhonghua Zhong Liu Za Zhi 2002;24:388-90. [PubMed]
- Kawamura M, Izumi Y, Tsukada N, et al. Percutaneous cryoablation of small pulmonary malignant tumors under computed tomographic guidance with local anesthesia for nonsurgical candidates. J Thorac Cardiovasc Surg 2006;131:1007-13. [PubMed]
- Wang H, Littrup PJ, Duan Y, et al. Thoracic masses treated with percutaneous cryotherapy: initial experience with more than 200 procedures. Radiology 2005;235:289-98. [PubMed]
- Wolf FJ, Grand DJ, Machan JT, et al. Microwave ablation of lung malignancies: effectiveness, CT findings, and safety in 50 patients. Radiology 2008;247:871-9. [PubMed]
- Yamauchi Y, Izumi Y, Hashimoto K, et al. Percutaneous cryoablation for the treatment of medically inoperable stage I non-small cell lung cancer. PLoS One 2012;7:e33223. [PubMed]
- Dupuy DE. Treatment of medically inoperable non-small-cell lung cancer with stereotactic body radiation therapy versus image-guided tumor ablation: can interventional radiology compete? J Vasc Interv Radiol 2013;24:1139-45. [PubMed]
- Hiraki T, Tajiri N, Mimura H, et al. Pneumothorax, pleural effusion, and chest tube placement after radiofrequency ablation of lung tumors: incidence and risk factors. Radiology 2006;241:275-83. [PubMed]
- Kothary N, Heit JJ, Louie JD, et al. Safety and efficacy of percutaneous fiducial marker implantation for image-guided radiation therapy. J Vasc Interv Radiol 2009;20:235-9. [PubMed]
- Dupuy DE, Mayo-Smith WW, Abbott GF, et al. Clinical applications of radio-frequency tumor ablation in the thorax. Radiographics 2002;22:S259-69. [PubMed]
- Sofocleous CT, May B, Petre EN, et al. Pulmonary thermal ablation in patients with prior pneumonectomy. AJR Am J Roentgenol 2011;196:W606-12. [PubMed]
- Ambrogi MC, Fanucchi O, Lencioni R, et al. Pulmonary radiofrequency ablation in a single lung patient. Thorax 2006;61:828-9. [PubMed]
- Skonieczki BD, Wells C, Wasser EJ, et al. Radiofrequency and microwave tumor ablation in patients with implanted cardiac devices: is it safe? Eur J Radiol 2011;79:343-6. [PubMed]
- Pua BB, Thornton RH, Solomon SB. Radiofrequency ablation: treatment of primary lung cancer. Semin Roentgenol 2011;46:224-9. [PubMed]
- Crabtree TD, Denlinger CE, Meyers BF, et al. Stereotactic body radiation therapy versus surgical resection for stage I non-small cell lung cancer. J Thorac Cardiovasc Surg 2010;140:377-86. [PubMed]
- Dupuy DE, Shulman M. Current status of thermal ablation treatments for lung malignancies. Semin Intervent Radiol 2010;27:268-75. [PubMed]
- Dupuy DE. Image-guided thermal ablation of lung malignancies. Radiology 2011;260:633-55. [PubMed]
- Organ LW. Electrophysiologic principles of radiofrequency lesion making. Appl Neurophysiol 1976-1977;39:69-76. [PubMed]
- Nahum Goldberg S, Dupuy DE. Image-guided radiofrequency tumor ablation: challenges and opportunities--part I. J Vasc Interv Radiol 2001;12:1021-32. [PubMed]
- Ahmed M, Liu Z, Afzal KS, et al. Radiofrequency ablation: effect of surrounding tissue composition on coagulation necrosis in a canine tumor model. Radiology 2004;230:761-7. [PubMed]
- Oshima F, Yamakado K, Akeboshi M, et al. Lung radiofrequency ablation with and without bronchial occlusion: experimental study in porcine lungs. J Vasc Interv Radiol 2004;15:1451-6. [PubMed]
- Morrison PR, vanSonnenberg E, Shankar S, et al. Radiofrequency ablation of thoracic lesions: part 1, experiments in the normal porcine thorax. AJR Am J Roentgenol 2005;184:375-80. [PubMed]
- Lu DS, Raman SS, Limanond P, et al. Influence of large peritumoral vessels on outcome of radiofrequency ablation of liver tumors. J Vasc Interv Radiol 2003;14:1267-74. [PubMed]
- Alexander ES, Dupuy DE. Lung Cancer Ablation: Technologies and Techniques. Semin intervent Radiol 2013;30:141-50.
- Simon CJ, Dupuy DE, Mayo-Smith WW. Microwave ablation: principles and applications. Radiographics 2005;25 Suppl 1:S69-83. [PubMed]
- Skinner MG, Iizuka MN, Kolios MC, et al. A theoretical comparison of energy sources--microwave, ultrasound and laser--for interstitial thermal therapy. Phys Med Biol 1998;43:3535-47. [PubMed]
- Brace CL, Hinshaw JL, Laeseke PF, et al. Pulmonary thermal ablation: comparison of radiofrequency and microwave devices by using gross pathologic and CT findings in a swine model. Radiology 2009;251:705-11. [PubMed]
- Lubner MG, Brace CL, Hinshaw JL, et al. Microwave tumor ablation: mechanism of action, clinical results, and devices. J Vasc Interv Radiol 2010;21:S192-203. [PubMed]
- Gage AA, Baust J. Mechanisms of tissue injury in cryosurgery. Cryobiology 1998;37:171-86. [PubMed]
- Izumi Y, Oyama T, Ikeda E, et al. The acute effects of transthoracic cryoablation on normal lung evaluated in a porcine model. Ann Thorac Surg 2005;79:318-22. [PubMed]
- Davalos RV, Mir IL, Rubinsky B. Tissue ablation with irreversible electroporation. Ann Biomed Eng 2005;33:223-31. [PubMed]
- Lee EW, Chen C, Prieto VE, et al. Advanced hepatic ablation technique for creating complete cell death: irreversible electroporation. Radiology 2010;255:426-33. [PubMed]
- Rubinsky B, Onik G, Mikus P. Irreversible electroporation: a new ablation modality--clinical implications. Technol Cancer Res Treat 2007;6:37-48. [PubMed]
- Dupuy DE, Aswad B, Ng T. Irreversible electroporation in a Swine lung model. Cardiovasc Intervent Radiol 2011;34:391-5. [PubMed]
- Deodhar A, Monette S, Single GW Jr, et al. Percutaneous irreversible electroporation lung ablation: preliminary results in a porcine model. Cardiovasc Intervent Radiol 2011;34:1278-87. [PubMed]
- Liu H, Steinke K. High-powered percutaneous microwave ablation of stage I medically inoperable non-small cell lung cancer: a preliminary study. J Med Imaging Radiat Oncol 2013;57:466-74. [PubMed]
- Zemlyak A, Moore WH, Bilfinger TV. Comparison of survival after sublobar resections and ablative therapies for stage I non-small cell lung cancer. J Am Coll Surg 2010;211:68-72. [PubMed]
- Simon TG, Beland MD, Machan JT, et al. Charlson Comorbidity Index predicts patient outcome, in cases of inoperable non-small cell lung cancer treated with radiofrequency ablation. Eur J Radiol 2012;81:4167-72. [PubMed]
- Belfiore G, Ronza F, Belfiore MP, et al. Patients’ survival in lung malignancies treated by microwave ablation: our experience on 56 patients. Eur J Radiol 2013;82:177-81. [PubMed]
- Lu Q, Cao W, Huang L, et al. CT-guided percutaneous microwave ablation of pulmonary malignancies: results in 69 cases. World J Surg Oncol 2012;10:80. [PubMed]
- Thomson KR, Cheung W, Ellis SJ, et al. Investigation of the safety of irreversible electroporation in humans. J Vasc Interv Radiol 2011;22:611-21. [PubMed]
- Steinke K, Sewell PE, Dupuy D, et al. Pulmonary radiofrequency ablation--an international study survey. Anticancer Res 2004;24:339-43. [PubMed]
- Nour-Eldin NE, Naguib NN, Saeed AS, et al. Risk factors involved in the development of pneumothorax during radiofrequency ablation of lung neoplasms. AJR Am J Roentgenol 2009;193:W43-8. [PubMed]
- Okuma T, Matsuoka T, Yamamoto A, et al. Frequency and risk factors of various complications after computed tomography-guided radiofrequency ablation of lung tumors. Cardiovasc Intervent Radiol 2008;31:122-30. [PubMed]
- Yamagami T, Kato T, Hirota T, et al. Pneumothorax as a complication of percutaneous radiofrequency ablation for lung neoplasms. J Vasc Interv Radiol 2006;17:1625-9. [PubMed]
- Sakurai J, Hiraki T, Mukai T, et al. Intractable pneumothorax due to bronchopleural fistula after radiofrequency ablation of lung tumors. J Vasc Interv Radiol 2007;18:141-5. [PubMed]
- Nomura M, Yamakado K, Nomoto Y, et al. Complications after lung radiofrequency ablation: risk factors for lung inflammation. Br J Radiol 2008;81:244-9. [PubMed]
- Lee JM, Jin GY, Goldberg SN, et al. Percutaneous radiofrequency ablation for inoperable non-small cell lung cancer and metastases: preliminary report. Radiology 2004;230:125-34. [PubMed]
- Sano Y, Kanazawa S, Gobara H, et al. Feasibility of percutaneous radiofrequency ablation for intrathoracic malignancies: a large single-center experience. Cancer 2007;109:1397-405. [PubMed]
- Nour-Eldin NE, Naguib NN, Mack M, et al. Pulmonary hemorrhage complicating radiofrequency ablation, from mild hemoptysis to life-threatening pattern. Eur Radiol 2011;21:197-204. [PubMed]
- Steinke K, King J, Glenn D, et al. Pulmonary hemorrhage during percutaneous radiofrequency ablation: a more frequent complication than assumed? Interact Cardiovasc Thorac Surg 2003;2:462-5. [PubMed]
- Vaughn C, Mychaskiw G 2nd, Sewell P. Massive hemorrhage during radiofrequency ablation of a pulmonary neoplasm. Anesth Analg 2002;94:1149-51. [PubMed]
- Yamakado K, Takaki H, Takao M, et al. Massive hemoptysis from pulmonary artery pseudoaneurysm caused by lung radiofrequency ablation: successful treatment by coil embolization. Cardiovasc Intervent Radiol 2010;33:410-2. [PubMed]
- Sakurai J, Mimura H, Gobara H, et al. Pulmonary artery pseudoaneurysm related to radiofrequency ablation of lung tumor. Cardiovasc Intervent Radiol 2010;33:413-6. [PubMed]
- Soh J, Toyooka S, Gobara H, et al. A case of delayed massive hemothorax caused by the rupture of a pulmonary artery pseudoaneurysm after radiofrequency ablation of lung tumors. Jpn J Clin Oncol 2012;42:646-9. [PubMed]
- Hiraki T, Gobara H, Mimura H, et al. Brachial nerve injury caused by percutaneous radiofrequency ablation of apical lung cancer: a report of four cases. J Vasc Interv Radiol 2010;21:1129-33. [PubMed]
- Matsui Y, Hiraki T, Gobara H, et al. Phrenic nerve injury after radiofrequency ablation of lung tumors: retrospective evaluation of the incidence and risk factors. J Vasc Interv Radiol 2012;23:780-5. [PubMed]
- Hiraki T, Gobara H, Kato K, et al. Bronchiolitis obliterans organizing pneumonia after radiofrequency ablation of lung cancer: report of three cases. J Vasc Interv Radiol 2012;23:126-30. [PubMed]
- Ghaye B, Bruyère PJ, Dondelinger RF. Nonfatal systemic air embolism during percutaneous radiofrequency ablation of a pulmonary metastasis. AJR Am J Roentgenol 2006;187:W327-8. [PubMed]
- Okuma T, Matsuoka T, Tutumi S, et al. Air embolism during needle placement for CT-guided radiofrequency ablation of an unresectable metastatic lung lesion. J Vasc Interv Radiol 2007;18:1592-4. [PubMed]
- Hiraki T, Mimura H, Gobara H, et al. Two cases of needle-tract seeding after percutaneous radiofrequency ablation for lung cancer. J Vasc Interv Radiol 2009;20:415-8. [PubMed]
- Yamakado K, Akeboshi M, Nakatsuka A, et al. Tumor seeding following lung radiofrequency ablation: a case report. Cardiovasc Intervent Radiol 2005;28:530-2. [PubMed]
- Abtin FG, Eradat J, Gutierrez AJ, et al. Radiofrequency ablation of lung tumors: imaging features of the postablation zone. Radiographics 2012;32:947-69. [PubMed]
- Beland MD, Wasser EJ, Mayo-Smith WW, et al. Primary non-small cell lung cancer: review of frequency, location, and time of recurrence after radiofrequency ablation. Radiology 2010;254:301-7. [PubMed]
- Yamamoto A, Nakamura K, Matsuoka T, et al. Radiofrequency ablation in a porcine lung model: correlation between CT and histopathologic findings. AJR Am J Roentgenol 2005;185:1299-306. [PubMed]
- Gadaleta C, Mattioli V, Colucci G, et al. Radiofrequency ablation of 40 lung neoplasms: preliminary results. AJR Am J Roentgenol 2004;183:361-8. [PubMed]
- Anderson EM, Lees WR, Gillams AR. Early indicators of treatment success after percutaneous radiofrequency of pulmonary tumors. Cardiovasc Intervent Radiol 2009;32:478-83. [PubMed]
- de Baère T, Palussière J, Aupérin A, et al. Midterm local efficacy and survival after radiofrequency ablation of lung tumors with minimum follow-up of 1 year: prospective evaluation. Radiology 2006;240:587-96. [PubMed]
- Yoo DC, Dupuy DE, Hillman SL, et al. Radiofrequency ablation of medically inoperable stage IA non-small cell lung cancer: are early posttreatment PET findings predictive of treatment outcome? AJR Am J Roentgenol 2011;197:334-40. [PubMed]
- Steinke K, King J, Glenn D, et al. Radiologic appearance and complications of percutaneous computed tomography-guided radiofrequency-ablated pulmonary metastases from colorectal carcinoma. J Comput Assist Tomogr 2003;27:750-7. [PubMed]
- Jin GY, Lee JM, Lee YC, et al. Primary and secondary lung malignancies treated with percutaneous radiofrequency ablation: evaluation with follow-up helical CT. AJR Am J Roentgenol 2004;183:1013-20. [PubMed]
- Okuma T, Okamura T, Matsuoka T, et al. Fluorine-18-fluorodeoxyglucose positron emission tomography for assessment of patients with unresectable recurrent or metastatic lung cancers after CT-guided radiofrequency ablation: preliminary results. Ann Nucl Med 2006;20:115-21. [PubMed]
- Ito N, Nakatsuka S, Inoue M, et al. Computed tomographic appearance of lung tumors treated with percutaneous cryoablation. J Vasc Interv Radiol 2012;23:1043-52. [PubMed]
- Hinshaw JL, Lee FT Jr, Laeseke PF, et al. Temperature isotherms during pulmonary cryoablation and their correlation with the zone of ablation. J Vasc Interv Radiol 2010;21:1424-8. [PubMed]
- Hinshaw JL, Littrup PJ, Durick N, et al. Optimizing the protocol for pulmonary cryoablation: a comparison of a dual- and triple-freeze protocol. Cardiovasc Intervent Radiol 2010;33:1180-5. [PubMed]
- Permpongkosol S, Nicol TL, Link RE, et al. Differences in ablation size in porcine kidney, liver, and lung after cryoablation using the same ablation protocol. AJR Am J Roentgenol 2007;188:1028-32. [PubMed]
- Callstrom MR, Charboneau JW, Goetz MP, et al. Image-guided ablation of painful metastatic bone tumors: a new and effective approach to a difficult problem. Skeletal Radiol 2006;35:1-15. [PubMed]
- Callstrom MR, Dupuy DE, Solomon SB, et al. Percutaneous image-guided cryoablation of painful metastases involving bone: multicenter trial. Cancer 2013;119:1033-41. [PubMed]
- Dupuy DE, Liu D, Hartfeil D, et al. Percutaneous radiofrequency ablation of painful osseous metastases: a multicenter American College of Radiology Imaging Network trial. Cancer 2010;116:989-97. [PubMed]
- Kojima H, Tanigawa N, Kariya S, et al. Clinical assessment of percutaneous radiofrequency ablation for painful metastatic bone tumors. Cardiovasc Intervent Radiol 2006;29:1022-6. [PubMed]
- Masuda E, Sista AK, Pua BB, et al. Palliative procedures in lung cancer. Semin intervent Radiol 2013;30:199-205.
- Gadaleta CD, Solbiati L, Mattioli V, et al. Unresectable lung malignancy: combination therapy with segmental pulmonary arterial chemoembolization with drug-eluting microspheres and radiofrequency ablation in 17 patients. Radiology 2013;267:627-37. [PubMed]
- Lindemayr S, Lehnert T, Korkusuz H, et al. Transpulmonary chemoembolization: a novel approach for the treatment of unresectable lung tumors. Tech Vasc Interv Radiol 2007;10:114-9. [PubMed]
- Vogl TJ, Herzog C, Zangos S, et al. Palliative treatment of primary lung tumors with transpulmonary chemoembolization (TPCE). Rofo 2007;179:300-7. [PubMed]
- Vogl TJ, Lehnert T, Zangos S, et al. Transpulmonary chemoembolization (TPCE) as a treatment for unresectable lung metastases. Eur Radiol 2008;18:2449-55. [PubMed]
- Vogl TJ, Wetter A, Lindemayr S, et al. Treatment of unresectable lung metastases with transpulmonary chemoembolization: preliminary experience. Radiology 2005;234:917-22. [PubMed]
- Monsky WL, Vien DS, Link DP. Nanotechnology development and utilization: a primer for diagnostic and interventional radiologists. Radiographics 2011;31:1449-62. [PubMed]
- Power S, Slattery MM, Lee MJ. Nanotechnology and its relationship to interventional radiology. Part II: Drug Delivery, Thermotherapy, and Vascular Intervention. Cardiovasc Intervent Radiol 2011;34:676-90. [PubMed]
- Power S, Slattery MM, Lee MJ. Nanotechnology and its relationship to interventional radiology. Part I: imaging. Cardiovasc Intervent Radiol 2011;34:221-6. [PubMed]
- Baumann P, Nyman J, Hoyer M, et al. Outcome in a prospective phase II trial of medically inoperable stage I non-small-cell lung cancer patients treated with stereotactic body radiotherapy. J Clin Oncol 2009;27:3290-6. [PubMed]
- Fakiris AJ, McGarry RC, Yiannoutsos CT, et al. Stereotactic body radiation therapy for early-stage non-small-cell lung carcinoma: four-year results of a prospective phase II study. Int J Radiat Oncol Biol Phys 2009;75:677-82. [PubMed]
- Lagerwaard FJ, Haasbeek CJ, Smit EF, et al. Outcomes of risk-adapted fractionated stereotactic radiotherapy for stage I non-small-cell lung cancer. Int J Radiat Oncol Biol Phys 2008;70:685-92. [PubMed]
- McGarry RC, Papiez L, Williams M, et al. Stereotactic body radiation therapy of early-stage non-small-cell lung carcinoma: phase I study. Int J Radiat Oncol Biol Phys 2005;63:1010-5. [PubMed]
- Onishi H, Araki T, Shirato H, et al. Stereotactic hypofractionated high-dose irradiation for stage I nonsmall cell lung carcinoma: clinical outcomes in 245 subjects in a Japanese multiinstitutional study. Cancer 2004;101:1623-31. [PubMed]
- Timmerman R, Paulus R, Galvin J, et al. Stereotactic body radiation therapy for inoperable early stage lung cancer. JAMA 2010;303:1070-6. [PubMed]
- Lanuti M, Sharma A, Willers H, et al. Radiofrequency ablation for stage I non-small cell lung cancer: management of locoregional recurrence. Ann Thorac Surg 2012;93:921-7; discussion 927-88. [PubMed]
- Donington J, Ferguson M, Mazzone P, et al. American College of Chest Physicians and Society of Thoracic Surgeons consensus statement for evaluation and management for high-risk patients with stage I non-small cell lung cancer. Chest 2012;142:1620-35. [PubMed]
- Crabtree T, Puri V, Timmerman R, et al. Treatment of stage I lung cancer in high-risk and inoperable patients: comparison of prospective clinical trials using stereotactic body radiotherapy (RTOG 0236), sublobar resection (ACOSOG Z4032), and radiofrequency ablation (ACOSOG Z4033). J Thorac Cardiovasc Surg 2013;145:692-9. [PubMed]